Inflammation, mitochondrial metabolism and nutrition: the multi-faceted progression of non-alcoholic fatty liver disease to hepatocellular carcinoma
Non-alcoholic fat liver disease (NAFLD) progression
When ectopic accumulation of fat in the liver is accompanied by cell death, inflammation and mitochondrial dysfunction, liver cells may switch from normal growth to an out-of-control state. One third of the population is now suffering NAFLD but estimates increase considerably in patients with consequences from comfortable lifestyles (1). This is because excessive food intake is currently responsible for the alarming increase in NAFLD prevalence and partially explains how sensitive is this condition to surgical procedures restricting the intake of nutrients. NAFLD is the common name for a wide range of phenotypes and it is a potentially serious condition that may progress from steatosis (NAFL) to steatohepatitis (NASH) to cirrhosis and hepatocellular carcinoma (HCC). This progression correlates with the severity of fibrosis but NAFLD can progress to HCC in the absence of cirrhosis, especially in an inflammatory context (2). Despite the clinical importance, the study of NAFLD progression is difficult not only because the issue is inherently complex, with multiple factors interacting simultaneously, but also because potential studies rely in invasive liver biopsy and the ensuing ethics. It is also hampered by the lack of validated non-invasive biomarkers or surrogates, effective medications and established preclinical models (3). Knowledge on basic mechanisms is also insufficient. Current efforts are directed towards understanding the heterogeneous outcomes of NAFLD and the assessment of dietary recommendations (4) but the rapid reversal of hepatic lesions after bariatric surgery may challenge some assumptions.
NAFLD and the selective immune cells death in carcinogenesis
The pace of innovative ideas is slow but Ma et al. (5) have investigated how inflammation and metabolic changes work together to promote hepatocarcinogenesis by feeding MYC oncogene transgenic mice (6) with NAFLD-inducing diets. In these models, NAFLD prompted the progression to HCC and, as expected, most immune cell subsets were increased. They found, however, an intriguingly selective loss of intrahepatic CD4+ T lymphocytes, which was apparently due to tumor-independent, NAFLD-dependent mechanisms. It is unclear why other cells escape this decline but “selectivity” is relevant because CD4+ T lymphocytes mediate in cancer progression (7). Indeed, when CD4+ antibody depletion was induced, these mice developed more hepatic tumor lesions than naive controls. Their findings strongly suggest a contributing role of CD4+ T lymphocytes in the development of HCC and confirm the association of chronic inflammation and decreased proportion of CD4+ cells with the HCC progression found in other models (8). Clinical relevance is also likely because adoptive cell therapies, using tumor-reactive T cells, have potential for cancer treatment: the presence of tumor-reactive CD4+ T helper cells can greatly enhance the anti-tumor activity of CD8+ cytotoxic T lymphocytes (9). There were no significant findings with regard to natural killer T (NKT) cells, illustrating the controversial disparities between human and mouse livers with respect to the role of NKT cell populations in NAFLD progression (10,11). Avoiding the loss of CD4+ cells may be a suggestive target.
Fatty acids, activation of immune cells and mitochondrial dysfunction
The relative accumulation in the liver of fatty acids contribute to NAFLD progression, modulate antigen presentation to immune cells and might help to explain this selective apoptosis in CD4+ T lymphocytes (5). Polyunsaturated fatty acids (PUFAs) are precursors of eicosanoids and their functions differ according to the chemical structure. They are classified as n-3 (omega-3) or n-6 (omega-6) depending on whether their first double bond is located on the third or sixth carbon from the terminal methyl group. n-6 PUFAS produce inflammatory prostaglandins or leukotrienes that contain two and four double bonds, respectively; by contrast those synthesized from n-3 fatty acids contain three and five double bonds, respectively, and have an anti-inflammatory role. Lipid levels were higher in CD4+ than in CD8+ T lymphocytes and, in the concentrations tested, linoleic acid (C18:2, n-6) was sufficient to accelerate CD4+ T lymphocytes death. Subsequent analysis showed that C18:2, n-6 selectively impaired electron transport chain, decreased mitochondrial membrane potential and increased mitochondrial reactive oxygen species (ROS) in CD4+ cells. Moreover, differentially elevated ROS levels were detected in CD4+ T lymphocytes ex vivo under NAFLD conditions and treatment with catalase or N-acetylcysteine (NAC) prevented C18:2, n-6-induced CD4+ T lymphocytes death in vitro (5). NAC, in vivo, also delayed NAFLD-promoted tumor development but data were less convincing. Collectively, these results may support mitochondrial dysfunction as a critical factor in promoting both NAFLD and hepatocarcinogenesis.
Oxidative stress and inflammation are inextricably linked and both contribute significantly to the pathogenesis of several diseases. Chronic inflammation is associated with oxidation, anti-inflammatory cascades are linked to decreased oxidation, increased oxidative stress triggers inflammation, and redox balance inhibits the inflammatory cellular response. The challenges are to understand how these relationships are part of the problem, how perturb pathways controlling metabolically driven chronic inflammatory states, and how affect the wound healing response to liver injury that results in liver cells death and loss of hepatic structure and function (12,13). Under these terms, finding solutions for NAFLD progression seems hopelessly complex.
So what? Weaknesses in nutritional therapies and a reference to bariatric surgery
How these data may help clinicians and patients? Essentially, liver biopsy studies may include the assessment of inflammatory cell infiltrate and the type of lipids in the diet should be carefully considered. However, clinical data to advance in the issue are scarce, sometimes contradictory and difficult to obtain and interpret during free living observations and different cultural and nutritional environments. In our hands, nutritional therapies have been anecdotally successful in maintaining long-term weight reduction and reversal of diabetes but bariatric surgery appears truly successful in the reversal of NAFLD progression of obese patients (Figure 1). Postoperative improvements in steatosis, NASH and fibrosis are out of proportion to weight loss. Perhaps it is time to seriously consider bariatric surgery as an experimental model to uncover molecular mechanisms and even as an intentional treatment option to halt NAFLD progression.
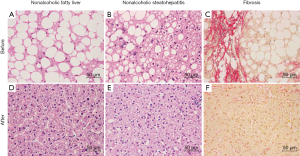
Selecting optimal dietary regimens from data in mice is unreliable because there is no sufficient concordance with human pathophysiology and the same regimen may lead to different outcomes depending on other manipulations in the animals (14). In particular, dietary factors used to induce NAFLD in mice deserve further consideration. We expect rapid effects feeding mice with methionine-choline-deficient (MCD) diets or other nutrient deviations but these are unlikely in humans and cause severe weight loss and liver atrophy. Contrarily, the use of high-fat (HF) and high cholesterol diet (15) may resemble human conditions but inter-laboratory variability is high and requires long-term feeding to produce fatty liver, obesity, NASH and fibrosis in mice. Additional models may be established with several genetic manipulations or dietary restrictions to track the progression of NAFLD but at the cost of adding further challenges to interpretation (16,17).
Other perceived disadvantages prevent extrapolation to humans. For instance, each dietary manipulation affects differently the overall metabolic state of the animals, and most have profound but unrealistic changes in mitochondrial function. Contrary to the response observed with MCD diets, the longitudinal assessment in HF diet-induced NAFLD reveals that the relative abundance of C18:2, n-6 remains constant. There is, however, a significant increase in oleic acid, which is a monounsaturated fatty acid (C18:1 cis-9), and a decrease in other PUFAs including arachidonic acid (C20:4, n-6), eicosapentanoic acid (EPA; C20:5, n-3) and docosahexaenoic acid (DHA; C22:6, n-3) (16). This effect is important because EPA and DHA are the precursors for resolvins and protectins, which bring about a programmed resolution of the inflammatory process (18). Likewise, n-3 fatty acids reduce cellular ROS production in humans and the association between a high n-6: n-3 ratio in both blood and liver and the severity of NAFLD is consistent (19). The family of 12/15 lipoxygenase enzymes also has relevance in NAFLD progression by controlling maturation of immune cells and implicating enzymatic lipid oxidation in the adaptive immune response (20). How to manipulate these particular aspects of human nutrition that are apparently inadequate to preserve liver healthy function? Some nutritional facts require attention. For example, in humans, palmitic acid (C16:0), a precursor to longer FAs, is the most common fatty acid in the liver because it is the first produced in response to excess carbohydrates. Linoleic acid (C18:2, n-6) represents 80−90% of dietary PUFA consumed in most countries and the most frequent dietary n-3 fatty acid is alpha-linolenic acid (C18:3, n-3). Although longer-chain n-3 fatty acids such as C20:5, n-3 and C22:6, n-3 can be synthesized from linolenic acid, the enzymatic reactions involved are inefficient and yield low amounts. A substantial increase requires major variations in common diets or expensive supplements.
Interventions on one-carbon metabolism?
Diets deficient in methionine and choline severely affect one-carbon metabolism, which support most metabolic processes (Figure 2). These alterations are particularly important in the liver and in humans are apparently the consequence of NAFLD rather than a possible cause. However, mechanistic roles in progression cannot be discarded. Intuitively, nutritional therapies, when indicated, should be designed to avoid these alterations or deficiencies. What we might expect in the liver of NAFLD patients is a significant depletion in methionine and an increase in S-adenosylmethionine (SAM), S-adenosylhomocysteine (SAH) and homocysteine. Serine, which is a substrate for both homocysteine remethylation and transsulfuration also decreases with a significant depletion in glutathione and reduced resistance to oxidative stress (15,21). Alterations in glutathione, choline, carnitine, serine and glycine, which are major sources of one-carbon units, compromise not only mitochondrial performance but also metabolic control (22). Perhaps more important for NAFLD progression is the fact that proliferative tissues are highly sensitive to changes in one-carbon metabolism, with relevance for the immune system and the development of cancer. The essential crosstalk between mitochondrial function and CD4+ T cell activation and the association with correct function of one-carbon and methionine metabolism has been recently highlighted (23). T lymphocytes are probably the immune components most affected by metabolic diseases and, when activated, undergo a metabolic switch regulating mitochondrial metabolism and immune responses. Effector T cells contain higher numbers of mitochondria than naive cells and a synchronized program of mitochondrial biogenesis during activation results in organelles with a new metabolic signature enriched for one-carbon metabolism. These effects are critical for survival of CD4+ T cells and likely relevant in the context of NAFLD (5,23). The massive induction of enzymes in the one-carbon metabolic pathway is surprising because enzymes within energy metabolism or fatty acid oxidation are the typical markers of mitochondrial biogenesis. Using mitochondrial one-carbon metabolism upon activation of CD4+ T cells, may also indicate that nutritional deficiencies may have a direct toxic effect in one-carbon flux and may help to predict novel therapeutic targets. For instance, serine is the most important donor of one-carbon units through the action of mitochondrial serine hydroxymethyltransferase (SHMT2). The synthesis of mitochondrial peptides also plays critical roles in metabolism and survival. Curiously, SHMT2 has an additional critical role in maintaining redox state via glutathione synthesis. Formate and NAC together may rescue SHMT2 deficient T cells from death illustrating the multifaceted role of mitochondria and inflammation in a nutritional context (23-25). Applying knowledge of individual nutrient requirements into clinical practice has the potential to improve outcomes and it is accepted that adequate dietary maintenance over the long term may support cellular integrity and health. However, the risk of adding bioactive elements to the diet of patients with NAFLD is too high in the absence of well-proven associations between their consumption and disease.
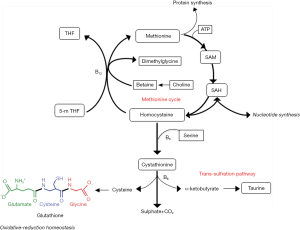
Conclusions
The association between NAFLD and HCC seems established but the specific sequence of events leading to HCC in the setting of NAFLD is still unresolved. Under a clinical point of view, we require integrative approaches to better understand the influence of potential consequences and targets derived from the study of dietary components. The combined impact of nutrient abundance and liver response in driving NAFLD progression represents a growing area of study. We envision a potential role of bariatric surgery as an experimental model but the challenge is to decipher the crosstalk between pathways that might interact in triggering liver damage and fibrosis. Ma et al. (5) highlights determinants of liver homeostasis that include inflammation and innate immune activation associated with mitochondrial metabolism, the way the liver handles metabolites that can accumulate during NAFLD, and the role of cell death pathways.
Acknowledgements
Funding: Current work in our laboratory is supported by grants from the Plan Nacional de I+D+I, Spain, Instituto de Salud Carlos III (Grant PI15/00285 co-founded by the European Regional Development Fund (FEDER)), the Agència de Gestió d’Ajuts Universitaris i de Recerca (SGR1227) and the Universitat Rovira I Virgili (JJ16376).
Footnote
Provenance: This is a Guest Editorial commissioned by Editor-in-Chief Yilei Mao (Department of Liver Surgery, Peking Union Medical College Hospital, Chinese Academy of Medical Sciences, Beijing, China).
Conflicts of Interest: The authors have no conflicts of interest to declare.
References
- Younossi ZM, Blissett D, Blissett R, et al. The Economic and Clinical Burden of Non-alcoholic Fatty Liver Disease (NAFLD) in the United States and Europe. Hepatology 2016. [Epub ahead of print]. [Crossref]
- Ertle J, Dechêne A, Sowa JP, et al. Non-alcoholic fatty liver disease progresses to hepatocellular carcinoma in the absence of apparent cirrhosis. Int J Cancer 2011;128:2436-43. [Crossref] [PubMed]
- Rodríguez-Gallego E, Guirro M, Riera-Borrull M, et al. Mapping of the circulating metabolome reveals α-ketoglutarate as a predictor of morbid obesity-associated non-alcoholic fatty liver disease. Int J Obes (Lond) 2015;39:279-87. [Crossref] [PubMed]
- Hernández-Aguilera A, Fernández-Arroyo S, Cuyàs E, et al. Epigenetics and nutrition-related epidemics of metabolic diseases: Current perspectives and challenges. Food Chem Toxicol 2016;96:191-204. [Crossref] [PubMed]
- Ma C, Kesarwala AH, Eggert T, et al. NAFLD causes selective CD4(+) T lymphocyte loss and promotes hepatocarcinogenesis. Nature 2016;531:253-7. [Crossref] [PubMed]
- Shachaf CM, Kopelman AM, Arvanitis C, et al. MYC inactivation uncovers pluripotent differentiation and tumour dormancy in hepatocellular cancer. Nature 2004;431:1112-7. [Crossref] [PubMed]
- Hoenicke L, Zender L. Immune surveillance of senescent cells--biological significance in cancer- and non-cancer pathologies. Carcinogenesis 2012;33:1123-6. [Crossref] [PubMed]
- Rakhra K, Bachireddy P, Zabuawala T, et al. CD4(+) T cells contribute to the remodeling of the microenvironment required for sustained tumor regression upon oncogene inactivation. Cancer Cell 2010;18:485-98. [Crossref] [PubMed]
- Li K, Baird M, Yang J, et al. Conditions for the generation of cytotoxic CD4(+) Th cells that enhance CD8(+) CTL-mediated tumor regression. Clin Transl Immunology 2016;5:e95. [Crossref] [PubMed]
- Adler M, Taylor S, Okebugwu K, et al. Intrahepatic natural killer T cell populations are increased in human hepatic steatosis. World J Gastroenterol 2011;17:1725-31. [Crossref] [PubMed]
- Hua J, Ma X, Webb T, et al. Dietary fatty acids modulate antigen presentation to hepatic NKT cells in nonalcoholic fatty liver disease. J Lipid Res 2010;51:1696-703. [Crossref] [PubMed]
- Calvo N, Beltrán-Debón R, Rodríguez-Gallego E, et al. Liver fat deposition and mitochondrial dysfunction in morbid obesity: An approach combining metabolomics with liver imaging and histology. World J Gastroenterol 2015;21:7529-44. [Crossref] [PubMed]
- Camps J, Rodríguez-Gallego E, García-Heredia A, et al. Paraoxonases and chemokine (C-C motif) ligand-2 in noncommunicable diseases. Adv Clin Chem 2014;63:247-308. [Crossref] [PubMed]
- Joven J, Rull A, Ferré N, et al. The results in rodent models of atherosclerosis are not interchangeable: the influence of diet and strain. Atherosclerosis 2007;195:e85-92. [Crossref] [PubMed]
- Vinaixa M, Rodríguez MA, Rull A, et al. Metabolomic assessment of the effect of dietary cholesterol in the progressive development of fatty liver disease. J Proteome Res 2010;9:2527-38. [Crossref] [PubMed]
- Rull A, Geeraert B, Aragonès G, et al. Rosiglitazone and fenofibrate exacerbate liver steatosis in a mouse model of obesity and hyperlipidemia. A transcriptomic and metabolomic study. J Proteome Res 2014;13:1731-43. [Crossref] [PubMed]
- Asgharpour A, Cazanave SC, Pacana T, et al. A diet-induced animal model of non-alcoholic fatty liver disease and hepatocellular cancer. J Hepatol 2016;65:579-88. [Crossref] [PubMed]
- Spite M, Clària J, Serhan CN. Resolvins, specialized proresolving lipid mediators, and their potential roles in metabolic diseases. Cell Metab 2014;19:21-36. [Crossref] [PubMed]
- Arendt BM, Comelli EM, Ma DW, et al. Altered hepatic gene expression in nonalcoholic fatty liver disease is associated with lower hepatic n-3 and n-6 polyunsaturated fatty acids. Hepatology 2015;61:1565-78. [Crossref] [PubMed]
- Rothe T, Gruber F, Uderhardt S, et al. 12/15-Lipoxygenase-mediated enzymatic lipid oxidation regulates DC maturation and function. J Clin Invest 2015;125:1944-54. [Crossref] [PubMed]
- Pacana T, Cazanave S, Verdianelli A, et al. Dysregulated Hepatic Methionine Metabolism Drives Homocysteine Elevation in Diet-Induced Nonalcoholic Fatty Liver Disease. PLoS One 2015;10:e0136822. [Crossref] [PubMed]
- Noland RC, Koves TR, Seiler SE, et al. Carnitine insufficiency caused by aging and overnutrition compromises mitochondrial performance and metabolic control. J Biol Chem 2009;284:22840-52. [Crossref] [PubMed]
- Ron-Harel N, Santos D, Ghergurovich JM, et al. Mitochondrial Biogenesis and Proteome Remodeling Promote One-Carbon Metabolism for T Cell Activation. Cell Metab 2016;24:104-17. [Crossref] [PubMed]
- Ducker GS, Chen L, Morscher RJ, et al. Reversal of Cytosolic One-Carbon Flux Compensates for Loss of the Mitochondrial Folate Pathway. Cell Metab 2016;23:1140-53. [Crossref] [PubMed]
- Cobb LJ, Lee C, Xiao J, et al. Naturally occurring mitochondrial-derived peptides are age-dependent regulators of apoptosis, insulin sensitivity, and inflammatory markers. Aging (Albany NY) 2016;8:796-809. [Crossref] [PubMed]