Cytochrome P450 2E1 inhibition prevents hepatic carcinogenesis induced by diethylnitrosamine in alcohol-fed rats
Introduction
Chronic alcohol abuse is a major risk factor for hepatocellular carcinoma in the United States and it has been estimated that 32-45% of all cases of hepatocellular carcinoma are due to alcohol consumption (1). Carcinogenesis is a process comprised of three main stages: initiation, promotion, and progression. Initiation is rapid and occurs with high frequency in the acute setting (with exposure to a carcinogen). Promotion is a long-term process and requires chronic exposure to a tumor-promoting environment, such as alcohol-induced oxidative stress, inflammation and/or nutritional imbalances. This injury can elicit a wide spectrum of cellular responses ranging from proliferation to growth arrest. Aside from oxidative stress, the causal relationship between inflammation and tumorigenesis has also been implicated in a variety of chronic inflammatory diseases. The pro-inflammatory cytokine tumor necrosis factor-alpha (TNF-α) is reported to be significantly increased in alcoholic patients (2,3). A previous study demonstrated reduced rates of preneoplastic lesions and liver tumor formation in TNF-α receptor knock-out mice (4), suggesting an important role of TNF-α signaling in hepatocarcinogenesis. In addition, nuclear factor-κB (NF-κB), an important transcriptional factor regulated by TNF-α in hepatocytes, is shown to be closely associated with liver neoplastic progression, mediating transcriptional regulation of multiple genes involved in cellular transformation, proliferation, and survival (5). Recently, we have shown that massive hepatic steatosis and alcoholic foamy degeneration with a mixed infiltrate of inflammatory cells and hepatocellular adenoma were detected in ethanol-fed rats after 10 months of treatment (6); but not in the control rats given the same dose of the DEN carcinogen. This clearly indicates the promoting effect of alcohol consumption on hepatic carcinogenesis (6).
Cytochrome P4502E1 (CYP2E1)-dependent microsomal ethanol oxidizing system, an important pathway of ethanol metabolism in the liver, is induced by chronic ethanol consumption (7). The induction of CYP2E1 by chronic alcohol intake results in an enhanced activation of procarcinogens to carcinogens (8), induction of oxidative stress (9), and release of inflammatory cytokines TNF-α, IL-6, and IL-1 (10-13), which may stimulate carcinogenesis (9). CYP2E1 knockout mice are shown to develop less oxidative stress (14), less oxidized DNA adducts (15) and less diethylnitrosamine (DEN)-induced hepatic tumors (16), as compared with wild-type mice. It has been shown that treatment with CYP2E1 inhibitors, such as chlormethiazole (CMZ) (17,18), protects against ethanol-induced liver injury (12,19-22). CMZ treatment significantly reduces ethanol-induced hepatic cytokine expression in ethanol-treated rats (12), decreases macrovesicular fat accumulation, and inhibits oxidative stress in mice fed ethanol (21). Furthermore, CMZ was shown to block the generation of ethanol-DNA adducts by 70%~90% in ethanol-treated cells overexpressing human CYP2E1 (22). However, direct evidence that CYP2E1 inhibition plays a role in ethanol-promoted hepatocarcinogenesis in vivo is still lacking.
In the present study, using CMZ as an inhibitor of CYP2E1, we examined the action of CYP2E1 in carcinogen-initiated and ethanol-promoted hepatocarcinogenesis in short- and long-term ethanol-fed rats. We also investigated potential biological mechanisms by which CMZ could affect hepatic carcinogenesis by measuring liver tissue levels of molecular markers including key pro-inflammatory cytokine TNF-α, transcription factor NF-κB, lipidperoxidation products 4-hydroxynonenal (4-HNE), exocyclic etheno-DNA adducts, oxidative DNA damage marker 8-hydroxy-2'-deoxyguanosine (8-OHdG), and retinoid concentrations.
Methods
Animals, diet, and study groups
Male Sprague-Dawley rats (130-140 g; from Charles River Laboratories, Wilmington, MA) were used in this study. For the short-term (1 month) study, rats were distributed into three groups (n=6) by weight-matching: (I) control group (C); (II) ethanol-fed group (E); and (III) ethanol-fed and CMZ (100 mg/kg BW) supplemented group (E + CMZ). In the long-term study (10 months), rats were divided into four groups (n=6): C, E, E + Low CMZ (10 mg/kg BW), and E + high CMZ (100 mg/kg BW). All ethanol-fed groups were fed the Lieber-Decarli liquid diet (Dyets Inc., Bethlehem, PA) containing 36% of total calories as ethanol, yielding a concentration of 6.2% (vol/vol). Ethanol was gradually introduced into experimental diets over a 7-day period before providing animals with the final concentration. In the control diet, ethanol was replaced by an isocaloric amount of maltodextrin (Purina Test diets, Richmond, IN, USA). Both diets contained 18% of total calories as protein and 35% as fat; 47% of total calories were provided from carbohydrates in the control diet, whereas 11% of total calories were from carbohydrates in the ethanol diet. For CMZ supplementation, CMZ was dissolved in 95% ethanol and added directly into the liquid diet. Since the liquid diet provides physiological amounts of fluid, extra water was not given.
For the long-term study, a low-dose diethylnitrosamine (DEN) (Sigma, St. Louis, MO) was given as an intraperitoneal injection of 20 mg/kg body weight one week before the ethanol feeding with or without CMZ began. While our recent study (6) has shown that this DEN treatment with chronic ethanol consumption over a ten-month period led to the development of hepatocellular adenoma in the DEN-initiated rats, we observed significant declines in preneoplastic placental form of glutathione S-transferase (p-GST) positive altered hepatocellular foci (AHF) in rodent livers after one month (Ye, Q., Chavez, P., and Wang, X.D., unpublished data) and 6- and 10-months of alcohol-feeding (6). In order to investigate the inhibitory effect of CMZ on ethanol-induced CYP2E1 on p-GST foci formation and inflammation in the short-term study, we injected rats with DEN (20 mg/kg i.p.) three weeks after ethanol feeding with or without CMZ treatment. Body weights were recorded weekly. All rats were terminally exsanguinated at the end of the study under AErrane® (Fort Dodge Animal Health, Fort Dodge, IO, USA) anesthesia. Liver tissues were collected, frozen under liquid nitrogen, and stored at -80 °C for further analysis. All animals were maintained in an American Association for the Accreditation of Laboratory Animal Care accredited facility, in an environmentally controlled atmosphere (temperature, 23 °C, 45% relative humidity) with 15 air changes of 100% fresh filtered air per hour and a 12/12 h light/dark cycle. All animals were observed daily for clinical signs of illness. This project was approved by the Jean Mayer USDA Human Nutrition Research Center on Aging Animal Care and Use Committee.
Immunohistochemistry
The altered hepatic foci, a marker for preneoplastic lesions (23,24), were measured by immunostaining of placental glutathione-s-transferase (p-GST) in the liver. Hepatocyte proliferation was quantified by immunohistochemical analysis of proliferating cell nuclear antigen (PCNA) and Ki-67. Briefly, sections (5 µm thick) were cut from formalin-fixed and paraffin embedded liver samples. After a standard dehydration-rehydration procedure, liver sections were incubated with 0.3% H2O2 for 30 min to quench endogenous peroxidase activity. The sections were then heated using steamer for 20 min in 10 mM sodium citrate (pH 6.0) buffer to retrieve antigen. The routine biotin-streptavidin immunohistochemical method consisted of sequential incubations in goat or horse serum blocking solution, polyclonal anti-GST (Novocastra Laboratory, UK) monoclonal anti-PCNA (clone PC10, Dako Cooperation, Carpinteria, CA, USA) or monoclonal anti-Ki-67 (clone MIB-5, Dako Cooperation), biotinylated goat anti-rabbit or horse anti-mouse IgG, and streptavidin conjugated to a horseradish peroxidase label. The slides were developed with a diaminobenzidine substrate and counterstained with hematoxylin. The sections were examined under light microscopy by two blinded independent investigators. For PCNA staining, a total of 20 randomly selected fields were screened and PCNA positive cells with dark brown nuclear were expressed as PCNA (+) per 100 hepatocytes. For Ki-67 staining, we counted the number of Ki-67 positive cells with dark brown nuclear in the entire slide, and Ki-67 positive cells were expressed as Ki-67(+) per cm2. For p-GST staining, we counted the number of animals that had p-GST-positive foci in each group.
Western blotting
Whole cell protein extraction for measuring CYP2E1, cyclin D1, and cleaved-caspase-3 protein levels, and nuclear extraction for measuring NF-κB p65 were prepared as previously described (25,26). Approximately 40 g protein extracts were resolved by SDS-PAGE and transferred onto Immobilon-P membranes (Millipore Corp., Bedford, MA). The membranes were blocked with 5% non-fat milk in TBST buffer and incubated with primary antibodies against CYP2E1 (Millipore Corp., Bedford, MA), NF-κB p65 (Santa Cruz Biotechnology, Inc., Santa Cruz, CA), cyclinD1 (Santa Cruz Biotechnology, Inc., Santa Cruz, CA), cleaved-caspase-3 (Cell Signaling Technology, Inc., Danvers, MA), or GAPDH (Millipore Corp., Bedford, MA). Membranes were then incubated with the secondary antibodies against rabbit or mouse (Bio-Rad Laboratory, Hercules, CA). The blots were then developed using a SuperSignal West Pico Chemiluminescent Substrate Kit (Pierce, Rockford, IL). The protein levels were analyzed with an imaging densitometer (Bio-Rad, Hercules, CA) using GAPDH as a loading control.
Real-time PCR analysis for hepatic TNF-α mRNA
Total RNA was extracted using TriPure reagent (Roche Applied Science, Indianapolis, IN). cDNA was generated with M-MLV reverse transcriptase (Invitrogen) as indicated in the manufacturer’s manual. Real-time PCR reactions were performed on an Applied Biosystems 7000 sequence detection system using Platinum SYBR Green qPCR Kit (Invitrogen) according to the manufacturer’s instructions. Sequences for PCR primers are as follows: TNF-α, 5'-CCAGACCCTCACACTCAGATCA-3' and 5'-TCCGCTTGGTGGTTTGCTA-3', GAPDH, 5'-AGTGCCAGCCTCGTCTCATAG-3' and 5'-CCTTGACTGTGCCGTTGAACT-3'. Quantification of gene expression was normalized to the levels of GAPDH and then calculated by reference to the average values for the control group using the comparative Ct method. For each sample and each gene, PCR reactions were carried out in duplicate and repeated twice.
Histopathology
Liver samples were fixed with 10% buffered formalin and embedded in paraffin wax. 5 µm sections were stained with hematoxylin and eosin (H&E). The slides were evaluated microscopically for the presence of tumors according to the criteria proposed by the World Health Organization (WHO)/International Agency for Research on Cancer (IARC) (27). The sections were photographed and examined by three independent investigators blinded to the treatment groups.
Immunohistochemical detection of etheno-DNA adducts
Staining on liver tissue was performed using the method developed in our laboratory (28,29). Formalin fixed slides were dipped in PBS for 10 minutes, then placed in 0.3% H2O2 in absolute methanol for 10 minutes to quench endogenous peroxidase. Slides were incubated with proteinase K (10 microg/mL) (Roche, Mannheim, Germany) in double distilled H2O at room temperature for 10 minutes to remove histone and nonhistone proteins from DNA, increasing antibody accessibility. After washing with PBS, slides were treated with 20 microg/mL ribonuclease (RNAse; Roche, Mannheim, Germany) (heated for 10 minutes at 80 °C to inactivate DNAse) at 37 °C for 1 hour to prevent antibody binding to RNA adducts, and then washed in PBS. To denature DNA, 4 N HCl was used for 5 minutes at room temperature and subsequently rinsed in PBS and double distilled water. The pH was neutralized with 50 mM Trisbase buffer, pH 7.4 for 5 minutes at room temperature. Nonspecific binding sites were blocked with 8% bovine serum albumin, 2% horse serum, 0.05% Tween, and 0.05% Triton X-100 for 20 minutes at room temperature. A cross adsorbed horse anti-mouse antibody, conjugated to biotin (Vector Laboratories, Burlingame, CA) was employed to prevent unspecific binding to endogenous rat immunoglobulin. Slides were incubated with the primary monoclonal antibody EM-A-1 against edA (provided by Drs. P.Lorenz and M. Rajewsky, University of Essen, Essen, Germany, at a dilution of 1:20 at 4 °C overnight (29). After washing with PBS, the antibody detection was performed using the Vectastain Elite ABC kit (Vector Laboratories, Burlingame, CA) according to the manufacturer’s protocol. Diaminobenzidine was used as a chromogen to visualize the reaction. The reaction was stopped after 5 minutes with H2O. Slides was counterstained with 4',6'-diamidino-2-phenylindole and mounted with Kaiser’s glycerin-gelatin. All slides were subjected to the same standard conditions. Negative controls were performed by omitting the primary antibody.
Immunohistochemical staining for protein-bound 4-HNE
Formaldehyde fixed sections were treated with 0.5% H2O2 in absolute methanol for 10 minutes to quench endogenous peroxidase activity. Thereafter, sections were incubated for 2 hours at room temperature with the primary antibody (rabbit anti-4HNE, 1:500, Alexis, Loerrach, Germany) and 5% normal serum to block nonspecific binding. Vectastain Lite ABC kit (Vectastain Laboratories, Burlingame, CA) was used for detection according to the manufacturer’s protocol. Staining was developed by incubating the sections for 5 minutes in diaminobenzidine. Sections were counterstained with hematoxylin and mounted in Aquatex mounting medium. Negative controls were performed by omitting the primary antibody.
Immunohistochemical staining for 8-OHdG
Formalin fixed liver sections were stained using the method developed by Yaborough et al. (30). After antigen retrieval, the slides were dipped in phosphate-buffered saline (PBS) for 10 minutes and then placed in 0.3% H2O2 in absolute methanol for 20 minutes to quench endogenous peroxidase. After washing with PBS, slides were treated with 10 µg/mL RNase A (EMD Chemicals, San Diego, CA92121, USA) (heated for 12 minutes at 80 °C to inactivate DNase) at 37 for 1 hour to prevent antibody binding to RNA adducts and then washed in PBS. To denature DNA, cells were treated with 4 N HCI for 5 minutes at room temperature and subsequently rinsed in double distilled water and PBS. The pH was neutralized with 50 mM Tribase buffer, pH 7.4, for 5 minutes at room temperature. Non-specific binding sites were blocked with 10% bovine serum albumin (BSA), 4% normal horse serum, 0.05% Tween 20 and 0.05% Triton X-100 for 20 minutes at 37 °C. Slides were incubated for 1 hour at room temperature with the primary monoclonal antibody mouse-anti-8-OH-guanosine (at a dilution of 1:8,000, Abcam, Cambridge, CB4 0WN, UK) and 2% normal horse serum to block nonspecific binding. After washing with PBS, the antibody detection was performed using the Vectastain Elite ABC kit (Vector Laboratories, Burlingame, CA/USA) according to the manufacturer’s protocol (incubation with secondary antibody: Horse anti-Mouse IgG (H + L) 1:400 for 40 minutes at room temperature). Diaminobenzidine (DAB) was used as a chromogen to visualize the reaction. The reaction was stopped after 5 minutes with PBS. Slides were counterstained with hematoxylin for 8 minutes at room temperature. After washed with running tap water for 5 minutes, differentiated with 1% HCl/70% Ethanol for 10 seconds and washed with tap water for 5 minutes. Turn blue with 1% sodium bicarbonate for 20 minutes. Dehydrated through 70% ethanol for 2×3 minutes, 95% ethanol for 2×3 minutes, 100% ethanol for 2×3 minutes and clear in xylene for 2×5 minutes. Mounted with mounting medium (Roti-Histokitt II). All slides were subjected to the same standardized conditions. Negative controls were performed by omitting the primary antibody.
Imaging and semiquantitative analysis of etheno-DNA adducts, 4-HNE, and 8-OHdG
Representative pictures were taken at a magnification of 200× with a Leica Image Manager 50 (Leica, Solms, Germany) and analyzed using Image J software (Toronto, Western Research Institure, UK). The frequency of etheno-DNA and 8-OHdG positive stained nuclei was expressed as percentage of stained nuclei over the total number of cells counted. Staining intensity for 4-HNE was assessed according to the scale devised by Tsutsumi et al. (31).
High performance liquid chromatography
Liver sample preparation and a gradient Reverse Phase-HPLC was performed to assess the retinoid levels in liver homogenates, as described previously (32,33). Briefly, 50 µL of each sample were injected onto the HPLC column. The gradient procedure at a flow rate of 1 mL/min was as follows: 100% solvent A (acetonitrile:tetrahydrofurane:water, 50:20:30, vol/vol/vol, with 0.35% acetic acid and 1% ammonium acetate in water) for 6 minutes, followed by a 6-minute linear gradient to 50% solvent A and 50% solvent B (acetonitrile:tetrahydrofurane:water, 50:44:6, vol/vol/vol, with 0.35% acetic acid and 1% ammonium acetate in water), an 11-minute hold at 50% solvent A/50% solvent B, a 2-minute gradient to 100% solvent B, and a 10 minute hold at 100% solvent B, then a 10-minute gradient back to solvent A, and finally a 7-minute hold at 100% solvent A. In this HPLC system, retinoic acid, retinol, and retinyl acetate eluted at 2.5, 4.8, and 8.9 minutes, respectively. Individual retinoids were identified by co-elution with standards and absorption spectrum analysis. Retinoids were quantified relative to the internal standard (retinyl acetate) by determining the peak areas calibrated against known amounts of standards.
Statistical analysis
All results values are expressed as means ± standard error of mean (SEM). Group means were compared using one-way analysis of variance (ANOVA) with Fisher’s least significant difference (LSD) post-hoc procedure. A difference between groups was considered significant if P<0.05. Groups not sharing the same letter are significantly different.
Results
Effects of ethanol and CMZ on hepatic CYP2E1 expression
Ethanol feeding for 1 month significantly increased hepatic CYP2E1 protein levels by 2.6 fold, as compared with control (P<0.05); while CMZ supplementation inhibited ethanol-induced hepatic CYP2E1 protein expression by approximately 45% (P<0.05, E vs. E + CMZ) (Figure 1A). Ethanol feeding for 10 months also significantly increased CYP2E1 protein level, as compared with control (P<0.05). CMZ supplementation for 10 months at both doses (10 and 100 mg/kg BW) significantly blocked the ethanol-induced hepatic CYP2E1 protein levels (Figure 1B).
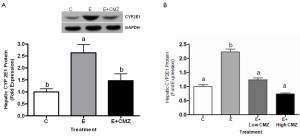
Effect of ethanol and CMZ on hepatocyte proliferation
Hepatocyte proliferation was assessed by immunohistochemical staining for PCNA (Figure 2A) and Ki-67 (Figure 2B) in rats fed ethanol for one month. Ethanol-feeding increased PCNA positive hepatocytes by 5.4 fold, while CMZ supplementation completely blocked ethanol-induced hepatocyte hyper-proliferation (Figure 2A). The result was confirmed by Ki-67 staining, another marker for cell proliferation. In the livers of rats fed a control diet, few hepatocytes were positively stained for Ki-67 (0.20±0.12 cells/cm2). The number of Ki-67 positive hepatocytes (2.25±0.69 cells/cm2) was dramatically increased by ethanol treatment (P<0.05, ethanol vs. control). CMZ supplementation significantly inhibited ethanol-induced hepatocyte proliferation, as no Ki-67 positive hepatocytes were detected in the livers of rats fed ethanol and supplemented with CMZ (Figure 2B).
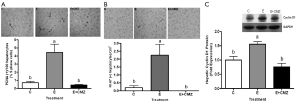
Hepatic cell proliferation was further evaluated by the expression of cyclin D1, a critical cell cycle regulator, using western blot. The cyclin D1 protein level was increased by about 50% in the liver tissues of ethanol-fed rats, as compared with rats fed a control diet (P<0.05); while no difference in liver cyclin D1 levels was found between control rats and rats fed ethanol and supplemented with CMZ (Figure 2C).
Effects of ethanol and CMZ on hepatocyte apoptosis in rat liver
Hepatic cell apoptosis was evaluated by the levels of cleaved-caspase-3 using western blot in rats fed ethanol for one month. Ethanol-feeding resulted in a 61% reduction in cleaved-caspase-3 protein levels in the rat livers (P<0.05, ethanol vs. control). CMZ supplementation in ethanol-fed rats increased the amount of cleaved-caspase-3 protein in liver tissues; however, the difference was not significant (Figure 3).
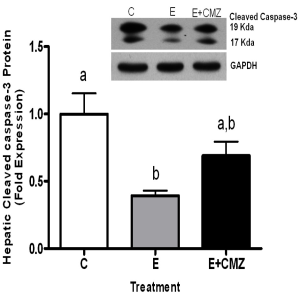
Effects of ethanol and CMZ on hepatic NF-κB activation and TNF-α expression
Hepatic NF-κB activation was assessed by nuclear distribution of NF-κB p65 subunit in rats fed ethanol for one month. Nuclear p65 protein levels were significantly increased in the liver of ethanol-fed rats (2.0-fold increase, as compared with control rats, P<0.05). In contrast, nuclear p65 levels in the livers of ethanol-fed and CMZ-supplemented rats were approximately 75% lower as compared with ethanol-fed rats (P<0.05) (Figure 4A).
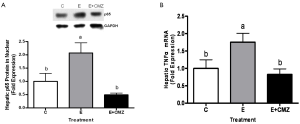
Hepatic TNF-α mRNA expression was measured by quantitative PCR. Ethanol feeding significantly stimulated hepative TNF-α mRNA expression (75% increase as compared with control, P<0.05); while CMZ supplementation inhibited ethanol-increased TNF-α expression (53% inhibition, as compared with ethanol-fed group, P<0.05) (Figure 4B).
Effects of ethanol and CMZ on the development of hepatic p-GST positive foci and the incidence of hepatocellular adenoma
Development of hepatic pre-neoplastic lesion in DEN-treated rats after 1 month of ethanol exposure was evaluated by the presence of p-GST positive foci in the livers. While no p-GST positive foci were found in all 6 rats fed a control diet, 4 out of 5 ethanol-fed rats developed hepatic p-GST positive foci (Figure 5). In CMZ and ethanol-fed group, p-GST positive foci were only found in the livers of one out of six rats (Figure 5).
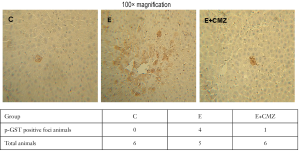
Development of hepatocellular adenoma in DEN-treated rats after 10 months of ethanol exposure with or without CMZ treatment was further examined. Without CMZ treatment, hepatocellular adenoma was detected in four out of five ethanol-fed rats after 10 months of treatment (Figure 6). None of ethanol-fed rats in groups treated with low- (10 mg/kg BW) or high- (100 mg/kg BW) doses of CMZ had hepatocellular adenoma after 10-month treatment period. Control rats given the same dose of DEN without ethanol did not develop hepatocellular adenoma at 10 months.
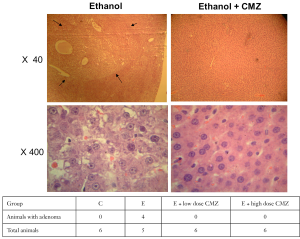
Effects of ethanol and CMZ on DNA adduct formation, 4-HNE and 8-OHdG positively stained nuclei in DEN-treated rats after 10 months of treatment
Chronic ethanol feeding did not significantly affect the generation of exocyclic etheno-DNA adducts in the liver [15.0±5.8% (C) vs. 16.0±8.9% (E) positively stained nuclei], while the administration of CMZ decreased the adduct formation, which, however was not statistically significant [16.0±8.9% (E) vs. 17.0±7.6% (E + Low CMZ) vs. 12.0±4.5% (E + High CMZ) positively stained nuclei]. This was paralleled by 4-HNE binding to protein. Again, no significant difference occurred between controls, ethanol fed rats and ethanol fed rats with CMZ [1.2±0.4 (C) vs. 1.2±0.5 (E) vs. 1.0±0.5 (E + High CMZ) score units]. In contrast, ethanol significantly increased 8-OHdG formation [11.7±4.1 (C) vs. 24±5.5 (E) positively stained nuclei, P<0.05] while the administration of CMZ significantly decreased 8-OHdG positively stained nuclei [14.3±5.4 (E + Low CMZ) and 14.0±5.0 (E + High CMZ), as compared with the E group, respectively, P<0.05].
Effects of ethanol and CMZ on the hepatic retinoid concentrations in DEN-treated rats after 10 months of treatment
Ethanol feeding for 10 months significantly decreased the hepatic concentrations of retinol and retinoic acid, as compared to non-ethanol fed control rats (P<0.05) (Figure 7). Compared to ethanol-fed rats, CMZ treatment at two different doses (10- and 100-mg/kg bw) in ethanol-fed rats restored hepatic retinol concentrations. There were no significant differences in hepatic retinol concentrations among control rats vs. ethanol-fed rats with CMZ treatment (Figure 7). Similarly, hepatic retinoic acid concentrations in low- and high-CMZ treatment groups were significantly increased as compared to ethanol-fed rats without CMZ treatment, and were not significantly different as compared to control rats.
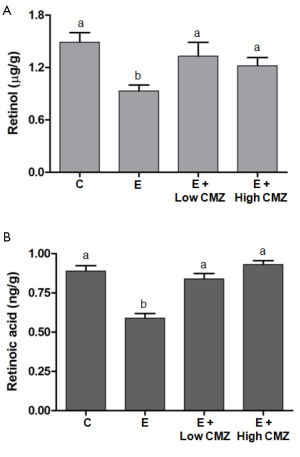
Discussion
This is the first study which reports a significant inhibition of alcohol-associated hepatocarcinogenesis by CYP2E1 inhibition. CMZ is a potent CYP2E1 inhibitor and its concomitant administration with alcohol resulted in a significant reduction of hepatic CYP2E1 in ethanol-fed rats at 1 as well as at 10 months of treatment. At the same time, we observed that hepatic p-GST positive foci, a marker for liver preneoplastic lesions, was detected in 4 out of 5 ethanol-fed rats but only 1 out of 6 ethanol-fed rats with CMZ treatment after one month. The same dose of DEN was given in rats fed a control diet, and none of them developed hepatic p-GST positive foci. Similarly, when the same dose of DEN was given in rats one week before the alcohol-feeding, hepatic CYP2E1 induction and adenomas were detected in the ethanol-fed rats, but were completely inhibited by CMZ after 10-months of treatment. These data clearly indicate that the inhibition of ethanol-induced CYP2E1 by CMZ can block carcinogen-initiated and ethanol-promoted hepatic carcinogenesis regardless the DEN given before or after alcohol-feeding. This finding is in accordance with data from a recent study, which showed lower incidence of DEN-initiated liver tumors in CYP2E1 knockout mice as compared with wild-type mice (16). This further indicates CYP2E1 as a key pathogenic factor in ethanol associated hepatocarcinogenesis.
Hepatocarcinogenesis is a complex process (34) in which inflammatory cytokines and oxidative stress (35) are of considerable importance (36). For example, infectious agents, stress signaling, and pro-inflammatory cytokines (37-39) activate NF-κB transcription factor, which mediates the production of various inflammation cytokines, such as IL-1, IL-6, and TNF-α (40), therefore promoting hepatic carcinogenesis. In addition to mediating the inflammatory response, NF-κB also regulates the expression of genes involved in many important processes during the development and progression of cancer, including cell proliferation and apoptosis (41). The present study showed an increased nuclear protein level of NF-κB p65 subunit and a higher TNF-α mRNA expression in the livers of ethanol-fed rats after DEN-injection, as compared with rats received DEN-injection but remained in non-ethanol diet. Since NF-κB promotes cell growth by regulating the expression of several genes in the cell cycle machinery, including cyclin D1 (42), it is important to note that the increased-nuclear accumulation of NF-κB protein and increased TNF-α expression by ethanol feeding was associated with increased hepatocyte proliferation markers and increased cyclin D1 protein expression in liver tissues. This association suggests that NF-κB activation may account for the increased hepatocyte proliferation. Consistent with our previous report (43), ethanol-feeding resulted in a significant reduction of apoptosis, as shown by decreased levels of cleaved caspase-3. The decreased apoptosis was associated with the activation of NF-κB in the livers of ethanol-fed rats. It is well known that NF-κB activation suppresses apoptosis, such that NF-κB targets various anti-apoptotic genes such as Bcl-XL, c-IAP, and c-Flip (39,44,45). These data indicate that the activation of NF-κB, followed by induction of TNF-α, increased cell proliferation and decreased apoptosis in the ethanol pre-fed rats, thus contributing to DEN-initiated hepatic carcinogenesis.
The present study strongly supports that ethanol-induced over-expressed CYP2E1 is a critical mediator, possibly through oxidative stress, for ethanol-induced inflammatory response, cell proliferation, and reduced apoptosis related to hepatocarcinogenesis. This notion was supported by our observation that the CMZ treatment in ethanol-fed rats significantly blocked the ethanol-induced CYP2E1 expression, NF-κB activation, TNF-α mRNA expression, and cell proliferation. Although we observed a strong inhibition of the NF-κB activation by CMZ in the livers of ethanol-fed rats, the CMZ supplementation in ethanol-fed rats only partially restored the apoptosis to normal level as compared with the control group. This could be due to other mechanisms, such as the involvement of the JunD gene which is induced by CYP2E1 and ethanol and forms part of the transcription factor complex AP-1 (46) that may contribute to ethanol-decreased apoptosis (47). Importantly, we showed that CMZ supplementation not only inhibited the development of p-GST-positive hepatic foci in the short term but also decreased the incidence of hepatocellular adenoma that were induced by DEN in ethanol fed rats in a long term study.
It has been well documented that chronic alcohol consumption generates lipid peroxidation products such as malondialdehyde and 4-hydroxynonenal (4-HNE), and the latter binds to adenosine and cytosine forming highly carcinogenic exocyclic etheno DNA adducts (48,49). These adducts have been identified in the liver of patients with alcoholic liver disease and other types of liver disease associated with inflammation and oxidative stress (22,50). In HepG2 cells overexpressing CYP2E1, we observed that these etheno-DNA adducts correlate significantly with CYP2E1 and 4-HNE and the adduct formation can be blocked by CMZ (22). Unexpectedly, in the present study, neither carcinogenic exocyclic etheno DNA adducts nor 4-HNE which is needed to form the adduct were changed among the treatment groups. In contrast, the oxidative DNA damage marker of 8-OHdG formation is clearly affected by ethanol and returned to almost normal with CMZ treatment. Since CYP2E1 induction forms reactive oxygen species (ROS) directly acting with DNA, it seems that the oxidative DNA damage reaction is favored compared to lipid peroxidation resulting in 4-HNE formation. The reason for this is not known, but may be related to the nitrosamine model used.
Retinoic acid is known to exert profound effects on cellular growth, differentiation, and apoptosis, thereby controlling alcohol-associated carcinogenesis (51). One of the observations in the present study is that the CMZ treatment not only prevented alcohol-promoted tumor formation but also restored reduced retinoic acid in the livers of rats after 10-months of treatment. These data were in agreement with our previous studies showing that alcohol-reduced levels of retinol and retinoic acid were prevented by CMZ, both in vitro and in vivo, indicating enhanced catabolism of vitamin A and retinoic acid by ethanol-induced CYP2E1 (19,20). This restoration of alcohol-reduced retinoic acid by CMZ provided further evidence supporting that the induction of hepatic CYP2E1 enzyme in chronic intermittent drinking is a factor in destroying retinol and retinoic acid. Further, the restoration of retinoic acid homeostasis by retinoic acid supplementation restored the normal status of both retinoid and MAPK signaling, thereby maintaining normal cell proliferation and apoptosis in the alcohol-fed animals (43,47). The observation that the CYP2E1 inhibitor can counteract the tumor-promoting action of ethanol by restoring normal hepatic levels of endogenous retinoic acid would have implications for the prevention of ethanol-promoted liver carcinogenesis, particularly regarding the detrimental effects of polar metabolites of vitamin A with alcohol feeding experiments (52). In summary, CYP2E1 inhibition may not only be an approach to prevent alcoholic liver disease, but also alcohol promoted hepatocarcinogenesis by restoring normal vitamin A levels. Therefore, the use of a safe CYP2E1 inhibitor without side effects may provide complementary or synergistic protective effects against alcohol-related cancer risk.
Acknowledgements
This work was a joint research program supported by NIH grant R01CA104932 and grants from National Natural Science Foundation of China (30730079) and grants of the Dietmar Hopp Foundation, the Manfred Lautenschläger Foundation, Heidelberg, Germany and a grant of the Gottlieb Daimler and Karl Benz Foundation, Ladenburg, Germany to HQ. The authors thank Camilla Peach for her assistance in the preparation of this manuscript.
Disclosure: The authors declare no conflict of interest.
References
- Morgan TR, Mandayam S, Jamal MM. Alcohol and hepatocellular carcinoma. Gastroenterology 2004;127:S87-96. [PubMed]
- Urbaschek R, McCuskey RS, Rudi V, et al. Endotoxin, endotoxin-neutralizing-capacity, sCD14, sICAM-1, and cytokines in patients with various degrees of alcoholic liver disease. Alcoholism, clinical and experimental research 2001;25:261-8. [PubMed]
- Bugianesi E. Non-alcoholic steatohepatitis and cancer. Clin Liver Dis 2007;11:191-207. [PubMed]
- Knight B, Yeoh GC, Husk KL, et al. Impaired preneoplastic changes and liver tumor formation in tumor necrosis factor receptor type 1 knockout mice. J Exp Med 2000;192:1809-18. [PubMed]
- He G, Karin M. NF-kappaB and STAT3 - key players in liver inflammation and cancer. Cell Res 2011;21:159-68. [PubMed]
- Chavez PR, Lian F, Chung J, et al. Long-term ethanol consumption promotes hepatic tumorigenesis but impairs normal hepatocyte proliferation in rats. J Nutr 2011;141:1049-55. [PubMed]
- Lieber CS. Cytochrome P-4502E1: its physiological and pathological role. Physiol Rev 1997;77:517-44. [PubMed]
- Seitz HK, Osswald BR. Effect of ethanol on procarcinogen activation. In: Watson RR. Alcohol and Cancered. Boca Raton: CRC press, 1992:167-78.
- Seitz HK, Stickel F. Molecular mechanisms of alcohol-mediated carcinogenesis. Nat Rev Cancer 2007;7:599-612. [PubMed]
- Earnest DL, Abril ER, Jolley CS, et al. Ethanol and diet-induced alterations in Kupffer cell function. Alcohol Alcohol 1993;28:73-83. [PubMed]
- Hansen J, Cherwitz DL, Allen JI. The role of tumor necrosis factor-alpha in acute endotoxin-induced hepatotoxicity in ethanol-fed rats. Hepatology 1994;20:461-74. [PubMed]
- Fang C, Lindros KO, Badger TM, et al. Zonated expression of cytokines in rat liver: effect of chronic ethanol and the cytochrome P450 2E1 inhibitor, chlormethiazole. Hepatology 1998;27:1304-10. [PubMed]
- Lin HZ, Yang SQ, Zeldin G, et al. Chronic ethanol consumption induces the production of tumor necrosis factor-alpha and related cytokines in liver and adipose tissue. Alcoholism, clinical and experimental research 1998;22:231S-7S. [PubMed]
- Lu Y, Wu D, Wang X, et al. Chronic alcohol-induced liver injury and oxidant stress are decreased in cytochrome P4502E1 knockout mice and restored in humanized cytochrome P4502E1 knock-in mice. Free Radic Biol Med 2010;49:1406-16. [PubMed]
- Bradford BU, Kono H, Isayama F, et al. Cytochrome P450 CYP2E1, but not nicotinamide adenine dinucleotide phosphate oxidase, is required for ethanol-induced oxidative DNA damage in rodent liver. Hepatology 2005;41:336-44. [PubMed]
- Kang JS, Wanibuchi H, Morimura K, et al. Role of CYP2E1 in diethylnitrosamine-induced hepatocarcinogenesis in vivo. Cancer Res 2007;67:11141-6. [PubMed]
- Hu Y, Mishin V, Johansson I, et al. Chlormethiazole as an efficient inhibitor of cytochrome P450 2E1 expression in rat liver. J Pharmacol Exp Ther 1994;269:1286-91. [PubMed]
- Gebhardt AC, Lucas D, Menez JF, et al. Chlormethiazole inhibition of cytochrome P450 2E1 as assessed by chlorzoxazone hydroxylation in humans. Hepatology 1997;26:957-61. [PubMed]
- Liu C, Chung J, Seitz HK, et al. Chlormethiazole treatment prevents reduced hepatic vitamin A levels in ethanol-fed rats. Alcoholism, clinical and experimental research 2002;26:1703-9. [PubMed]
- Liu C, Russell RM, Seitz HK, et al. Ethanol enhances retinoic acid metabolism into polar metabolites in rat liver via induction of cytochrome P4502E1. Gastroenterology 2001;120:179-89. [PubMed]
- Lu Y, Zhuge J, Wang X, et al. Cytochrome P450 2E1 contributes to ethanol-induced fatty liver in mice. Hepatology 2008;47:1483-94. [PubMed]
- Wang Y, Millonig G, Nair J, et al. Ethanol-induced cytochrome P4502E1 causes carcinogenic etheno-DNA lesions in alcoholic liver disease. Hepatology 2009;50:453-61. [PubMed]
- Sato K, Kitahara A, Satoh K, et al. The placental form of glutathione S-transferase as a new marker protein for preneoplasia in rat chemical hepatocarcinogenesis. Gann 1984;75:199-202. [PubMed]
- Kitahara A, Satoh K, Nishimura K, et al. Changes in molecular forms of rat hepatic glutathione S-transferase during chemical hepatocarcinogenesis. Cancer Res 1984;44:2698-703. [PubMed]
- Lian F, Smith DE, Ernst H, et al. Apo-10'-lycopenoic acid inhibits lung cancer cell growth in vitro, and suppresses lung tumorigenesis in the A/J mouse model in vivo. Carcinogenesis 2007;28:1567-74. [PubMed]
- Kim Y, Chongviriyaphan N, Liu C, et al. Combined antioxidant (beta-carotene, alpha-tocopherol and ascorbic acid) supplementation increases the levels of lung retinoic acid and inhibits the activation of mitogen-activated protein kinase in the ferret lung cancer model. Carcinogenesis 2006;27:1410-9. [PubMed]
- Mohr U. International Classification of Rodent Tumors. Part I: The Rat 10. Digestive System. ed. The International Agency for Research on Cancer, 1997.
- Frank A, Seitz HK, Bartsch H, et al. Immunohistochemical detection of 1,N6-ethenodeoxyadenosine in nuclei of human liver affected by diseases predisposing to hepato-carcinogenesis. Carcinogenesis 2004;25:1027-31. [PubMed]
- Yang Y, Nair J, Barbin A, et al. Immunohistochemical detection of 1,N(6)-ethenodeoxyadenosine, a promutagenic DNA adduct, in liver of rats exposed to vinyl chloride or an iron overload. Carcinogenesis 2000;21:777-81. [PubMed]
- Yarborough A, Zhang YJ, Hsu TM, et al. Immunoperoxidase detection of 8-hydroxydeoxyguanosine in aflatoxin B1-treated rat liver and human oral mucosal cells. Cancer Res 1996;56:683-8. [PubMed]
- Tsutsumi M, Lasker JM, Shimizu M, et al. The intralobular distribution of ethanol-inducible P450IIE1 in rat and human liver. Hepatology 1989;10:437-46. [PubMed]
- Wang XD, Liu C, Chung J, et al. Chronic alcohol intake reduces retinoic acid concentration and enhances AP-1 (c-Jun and c-Fos) expression in rat liver. Hepatology 1998;28:744-50. [PubMed]
- Luvizotto RA, Nascimento AF, Veeramachaneni S, et al. Chronic Alcohol Intake Up-regulates Hepatic Expressions of Carotenoid Cleavage Enzymes and Peroxisomal Proliferator-Activated Receptors in Rats. J Nutr 2010;140:1808-14. [PubMed]
- Dominguez-Malagón H, Gaytan-Graham S. Hepatocellular carcinoma: an update. Ultrastruct Pathol 2001;25:497-516. [PubMed]
- Tien Kuo M, Savaraj N. Roles of reactive oxygen species in hepatocarcinogenesis and drug resistance gene expression in liver cancers. Mol Carcinog 2006;45:701-9. [PubMed]
- Berasain C, Castillo J, Perugorria MJ, et al. Inflammation and liver cancer: new molecular links. Ann N Y Acad Sci 2009;1155:206-21. [PubMed]
- Janssen-Heininger YM, Poynter ME, Baeuerle PA. Recent advances towards understanding redox mechanisms in the activation of nuclear factor kappaB. Free Radic Biol Med 2000;28:1317-27. [PubMed]
- Kabe Y, Ando K, Hirao S, et al. Redox regulation of NF-kappaB activation: distinct redox regulation between the cytoplasm and the nucleus. Antioxid Redox Signal 2005;7:395-403. [PubMed]
- Karin M. Nuclear factor-kappaB in cancer development and progression. Nature 2006;441:431-6. [PubMed]
- Vanden Berghe W, Vermeulen L, Delerive P, et al. A paradigm for gene regulation: inflammation, NF-kappaB and PPAR. Adv Exp Med Biol 2003;544:181-96. [PubMed]
- Dolcet X, Llobet D, Pallares J, et al. NF-κB in development and progression of human cancer. Virchows Arch 2005;446:475-82. [PubMed]
- Joyce D, Albanese C, Steer J, et al. NF-kappaB and cell-cycle regulation: the cyclin connection. Cytokine Growth Factor Rev 2001;12:73-90. [PubMed]
- Chung J, Chavez PR, Russell RM, et al. Retinoic acid inhibits hepatic Jun N-terminal kinase-dependent signaling pathway in ethanol-fed rats. Oncogene 2002;21:1539-47. [PubMed]
- Beg AA, Baltimore D. An essential role for NF-kappaB in preventing TNF-alpha-induced cell death. Science 1996;274:782-4. [PubMed]
- Liu ZG, Hsu H, Goeddel DV, et al. Dissection of TNF receptor 1 effector functions: JNK activation is not linked to apoptosis while NF-kappaB activation prevents cell death. Cell 1996;87:565-76. [PubMed]
- Butura A, Nilsson K, Morgan K, et al. The impact of CYP2E1 on the development of alcoholic liver disease as studied in a transgenic mouse model. J Hepatol 2009;50:572-83. [PubMed]
- Chung J, Liu C, Smith DE, et al. Restoration of retinoic acid concentration suppresses ethanol-enhanced c-Jun expression and hepatocyte proliferation in rat liver. Carcinogenesis 2001;22:1213-9. [PubMed]
- el Ghissassi F, Barbin A, Nair J, et al. Formation of 1,N6-ethenoadenine and 3,N4-ethenocytosine by lipid peroxidation products and nucleic acid bases. Chem Res Toxicol 1995;8:278-83. [PubMed]
- Chung FL, Chen HJ, Nath RG. Lipid peroxidation as a potential endogenous source for the formation of exocyclic DNA adducts. Carcinogenesis 1996;17:2105-11. [PubMed]
- Nair J, Srivatanakul P, Haas C, et al. High urinary excretion of lipid peroxidation-derived DNA damage in patients with cancer-prone liver diseases. Mutat Res 2010;683:23-8. [PubMed]
- Wang XD. Alcohol, vitamin A, and cancer. Alcohol 2005;35:251-8. [PubMed]
- Dan Z, Popov Y, Patsenker E, et al. Hepatotoxicity of alcohol-induced polar retinol metabolites involves apoptosis via loss of mitochondrial membrane potential. FASEB J 2005;19:845-7. [PubMed]