The rate of muscle wasting in liver transplant recipients on waiting list: post-transplant outcomes and associated serum metabolite patterns
Highlight box
Key findings
• The rate of progression of sarcopenia is a strong prognostic indicator of post-liver transplantation (LT) mortality.
• Serum metabolite profiles of patients with rapid progression of sarcopenia most closely resemble derangements associated with chronic liver disease.
What is known and what is new?
• Sarcopenia at the time of LT is an established risk factor for mortality following LT, but most studies in this context have defined sarcopenia by one-time, static measurements.
• The aims of this study were (I) to determine the impact of the rate of muscle loss in waitlisted LT recipients on post-LT outcomes and (II) to identify patterns of serum metabolites associated with patients with more progressive sarcopenia.
What is the implication and what should change now?
• Metabolic perturbations identified herein suggest that nutritional interventions aimed at maintaining muscle mass may benefit liver transplant patients irrespective of the rate of sarcopenic progression.
Introduction
Sarcopenia is the significant loss of muscle mass as the result of normal aging or an underlying pathologic process (1). A strong link exists between sarcopenia and liver disease, with a prevalence between 40% and 70% in end-stage liver disease (ESLD) patients (2). Within the cirrhotic population, sarcopenia has been associated with increased hospitalizations, disease-related complications, and health care costs (3,4). Additionally, sarcopenia demonstrates significant influence on liver transplantation (LT) outcomes, contributing to increased waitlist mortality, post-transplant mortality, post-transplant infection, and length of post-operative hospitalization (5-7). While standard metrics [Model for End-Stage Liver Disease (MELD) and Child-Pugh scores] are utilized for prognostic and organ allocation purposes, studies have shown that these measures of liver dysfunction do not correlate with sarcopenia (8). Therefore, assessment for sarcopenia is important for identification of patients whose risk may not be adequately captured by conventional means.
Current hypotheses suggest sarcopenia in the cirrhotic population is the result of an imbalance between muscle synthesis and muscle breakdown (9). ESLD promotes a proteolytic state, providing substrate for gluconeogenesis as a way to compensate for impaired glycogen storage of the cirrhotic liver, and for use in ammonia detoxification (10). As a result, ESLD patients have lower levels of circulating branched-chain amino acids (BCAAs) as compared to healthy controls (11). Liver failure is also associated with an increase in circulating aromatic amino acids (AAAs) due to a decrease in AAA breakdown in the liver (12). The ratio of the concurrent decrease in circulating BCAAs and increase in AAAs is called Fischer’s index and is an indicator of liver dysfunction. Muscle breakdown may be further accentuated by increased levels of the pro-inflammatory cytokines tumor necrosis factor (TNF)-α, interleukin (IL)-1, and IL-6, which have been associated with upregulation of the ubiquitin-proteasome pathway (UPP) (13). Additionally, cirrhotic patients have been identified as having lower caloric intake and impaired absorption of fats and vitamins (10). Taken together, these observations suggest that the depletion of building block metabolites necessary for maintenance of skeletal muscle mass may explain the sarcopenia of liver disease. However, the wide range of muscle wasting observed in cirrhotic populations suggests that this process remains poorly understood (7).
The quantification of sarcopenia in patients is most effectively performed through imaging modalities. Dual-energy X-ray absorptiometry (DEXA) has been utilized in numerous studies examining age-related changes in body composition, however this technique is limited by anasarca or ascites commonly seen in liver patients (14). As an alternative, single slice axial computed tomography (CT) techniques have been identified as an accurate measure of skeletal muscle mass, visceral fat, and subcutaneous fat. These measurements have been subsequently validated as predictors of total body composition and nutritional status in the LT population (15-17). Studies performed using these methods have thus far been limited by utilizing one-time measurements of body composition, and few account for changes in lean muscle mass that occur over the course of a patient’s liver disease (18). We hypothesized that the rate of muscle wasting influenced post-LT outcomes more so than one time measurement of sarcopenia.
Here, utilizing serial CT scan assessments of sarcopenia, we demonstrate the importance of the rate of muscle wasting to LT outcomes. Additionally, with metabolomic analysis, we examine whether serum metabolites profiles in patients with relatively rapid progression of wasting differed from other patients. We present this article in accordance with the STROBE reporting checklist (available at https://hbsn.amegroups.com/article/view/10.21037/hbsn-23-645/rc).
Methods
Patient selection and clinical data collection
All liver transplant recipients between 2008 and 2018 were retrospectively identified within the prospectively maintained Indiana University Liver Transplant database (n=1,513). From this group, patients who underwent at least two CT scans within a period of 12 months and at least 4 months apart prior to transplantation, were identified (n=129). Of the 129 subjects who met these criteria, 61 patients were selected pursuant to available clinical follow-up. Demographic and clinical outcomes data was obtained from the electronic medical records. Additionally, 10 living-related kidney donor (LKD) patients were identified for use as healthy controls for metabolite analyses. All research was conducted in accordance with both the Declarations of Helsinki (as revised in 2013) and Istanbul. Analysis of patient data for this study was reviewed and approved by the Institutional Review Board of the Indiana University School of Medicine (IRB #1305011413) and individual consent for this retrospective analysis was waived.
Psoas muscle measurement and interpretation
The psoas muscle index (PMI) was utilized as a surrogate for sarcopenia as previously described (15,19). PMI is defined as the cross-sectional area of right and left psoas muscle at the level of L3/L4 relative to the patient’s height squared (cm2/m2). To obtain the psoas measurements, patients’ CT imaging was reviewed using Slice-O-Matic software. The area of right and left psoas muscles area was measured at the most superior aspect of L3/L4 using the freehand drawing function, and the areas were summed to obtain the total psoas muscle area. Total psoas area was normalized by each patient’s height squared to generate the PMI. To minimize human error in CT measurements, each image was analyzed by three individual research assistants who had been trained in interpreting abdominal CT images and Slice-O-Matic software. Research assistants were blinded to patients, their outcomes, and the measurements made by other assistants. Psoas area measurements were compared between reviewers. Any individual measurement that differed by >15% of the mean was re-examined until a consensus was reached. The mean of the three psoas measurements for each patient was used to calculate the PMI (17).
Median rate of progression was −0.5% change PMI/month. There was no difference in post-LT survival when using this cutoff to define median high wasting (HWmed) vs. median low wasting (LWmed) (Figure S1). Therefore, we performed an additional subset analysis of patients with extreme progression of sarcopenia, as defined as >1% decrease per month. Patients with >1% decrease in PMI per month were considered to be very HW (VHW) and patients with <1% decrease/month PMI change were considered to be LW.
Serum nuclear magnetic resonance (NMR) metabolomic analysis
As part of standard care, serum samples from all recipients and living donors are archived prior to transplant/donation at our institution. Serum from 36 LT recipients was available in sufficient quantity for this analysis. We elected to use the median change in PMI (ΔPMI) of the cohort (−0.5%/month) to delineate between HWmed and LWmed for metabolomics analysis. The final sample set included 15 patients in the HWmed group, 21 in the LWmed group, and 10 in the LKD group. We performed a similar subset analysis as well, comparing metabolite profiles in VHW patients against LW and LKD controls.
For NMR-based metabolomics, 100 mL aliquots of serum samples were diluted with 300 mL of deuterated phosphate buffer (pH 7.4). The solution was filtered through a 10 kDa molecular weight cutoff filter to remove proteins and lipoproteins. To the filtrate was added 60 mL of a Chenomx reference solution containing 5.0 mM, 2,2-dimethyl-2-silapentane-5-sulfonate-d6 (DSS-d6) sodium salt as an internal chemical shift and quantitation standard. The total volume of each sample was brought to 600 mL with deuterated phosphate buffer.
NMR data were acquired on a Bruker Avance III 700 MHz NMR spectrometer with a triple-resonance (TXI) probe operating at 25 ℃. Spectra were collected with a 1D NOESY pulse sequence covering 12 ppm. The spectra were digitized with 32,768 points during a 3.9-second acquisition time. The mixing time was set to 100 ms and the relaxation delay between scans was set to 2.0 seconds.
The data were processed using the Advanced Chemistry Development Spectrus Processor (version 2016.1, Toronto, Canada). The spectra were zero filled to 65,536 points, apodised using a 0.3 Hz decaying exponential function and fast Fourier transformed. Automated phase correction and linear baseline correction were applied to all samples. Metabolite concentrations were quantified using the Chenomx NMR Suite (version 8.2, Edmonton, Canada). The DSS-d6 was used as a chemical shift and quantification reference for all spectra and was set to a chemical shift of 0.00 and a concentration of 0.5 mM. Quantitative fitting of each spectrum was carried out in batch mode, followed by manual adjustment for some spectra to correct for errors arising from spectral overlap.
NMR lipoprotein analysis
As the liver plays a major role in lipoprotein metabolism, we measured a range of lipoprotein particle concentrations. Determination of lipoprotein particle subfractions by NMR was assessed using the NMR-based platform at LipoScience (now LabCorp, Morrisville, NC, USA). Lipoprotein particle numbers and sizes were determined using a version of the LP2 algorithm modified for murine plasma (20). A total of 21 lipoprotein subclasses were measured.
Statistical analysis
Patient demographic and outcomes data is expressed as medians with interquartile ranges (IQRs) unless otherwise specified. Student’s t-test, analysis of variance (ANOVA), chi-squared, and nonparametric hypothesis testing was employed as relevant. Metabolomics data was analyzed using Viime using ANOVA with Tukey post-hoc analysis (21). Volcano plots were generated in R. All hypotheses were tested at a significance level of 0.05 using two-tailed tests.
Results
Patient demographics and clinical outcomes—median ΔPMI cutoff
In total, 61 patients were identified with >1 CT scan obtained prior to LT. Median time between CT scans was 8.3 months (IQR, 4.7, 11 months). Thirty patients were classified as HWmed and 31 patients as LWmed according to their rate of wasting as compared to the median of the study population. Demographic variables were similar between these two groups (Table S1). Importantly, there was no significant difference in post-LT survival outcomes. The 1-year patient and graft survival rates were statistically similar between HWmed and LWmed groups on Kaplan-Meier survival curve analysis (Figure S1: 86.7% vs. 93.5%, P=0.38).
Patient demographics and clinical outcomes—subgroup analysis (ΔPMI <−1%)
While using the median ΔPMI as a cutoff resulted in even cohorts, this classification did not identify any significant differences in post-transplant outcomes. We therefore sought to identify a ΔPMI cutoff that was associated with a difference in post-LT survival. We found that patients with a decrease in PMI of >1%/month had significantly worse 1-year graft and patient survival rates as compared to the rest of the study population (73.3% vs. 93.5%, P=0.03; Figure 1). After identifying this inflection point, we proceeded to perform a subset analysis. A group of 15 patients were classified as VHW and 31 patients as LW using these criteria. Demographic variables are summarized in Table 1. Patients in each group were of similar age, sex, and MELD score at time of transplant. Etiology of liver failure in each group was similar, as was the proportion of patients with hepatocellular carcinoma (HCC) on explant pathology. The proportion of VHW patients with pre-operative ascites or hepatic encephalopathy was significantly greater than in the LW group. Median ΔPMI was −2.0%/month in VHW and −0.15%/month in LW patients (P<0.001). Body mass index (BMI) (25.2 vs. 29.8 kg/m2, P=0.002) and PMI (4.2 vs. 6.4 cm2/m2, P=0.04) at time of transplant were significantly lower in the HW group. These patients also had significantly lower pre-transplant serum albumin levels as compared to the rest of the study (2.6 vs. 3.1 g/dL, P=0.03). Patients in the HW group spent significantly less time on the waitlist (26 vs. 68.5 days, P=0.03).
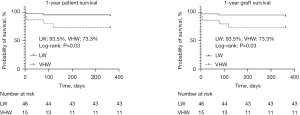
Table 1
Variables | Wasting group | P value | |
---|---|---|---|
VHW (n=15) | LW (n=46) | ||
Age at Txp (years) | 63 [61, 66] | 63.5 [61, 66] | 0.74 |
Sex (female) | 3 (20.0) | 13 (28.3) | 0.53 |
Race (Caucasian) | 13 (86.7) | 40 (87.0) | |
MELD at Txp | 22 [21, 29] | 21.5 [16.7, 23] | 0.08 |
Pre-LT GFR (mL/min) | >60 [43.3, >60] | >60 [47, >60] | 0.72 |
Etiology liver disease | 0.30 | ||
NASH | 6 (40.0) | 18 (39.1) | |
Alcoholic | 2 (13.3) | 12 (26.1) | |
Idiopathic | 5 (33.3) | 14 (30.4) | |
Hepatitis C | 2 (13.3) | 1 (2.2) | |
Malignancy | 0 | 1 (2.2) | |
History of ascites | 14 (93.3) | 31 (67.4) | 0.04* |
History of hepatic encephalopathy | 14 (93.3) | 31 (67.4) | 0.04* |
History of diabetes | 6 (40.0) | 23 (50.0) | 0.50 |
History of tobacco use | 11 (73.3) | 28 (60.9) | 0.38 |
Waitlist days | 26 [6, 72] | 68.5 [20.8, 122] | 0.03* |
HCC on explant pathology | 9 (60.0) | 17 (37.0) | 0.12 |
BMI at transplant (kg/m2) | 25.2 [21.7, 27.3] | 29.8 [26.4, 34] | 0.002* |
Initial PMI (cm2/m2) | 6.11 [4.9, 7.8] | 6.2 [4.2, 7.5] | 0.93 |
PMI at transplant (cm2/m2) | 4.2 [3.7, 6.3] | 6.4 [4.3, 7.5] | 0.04* |
ΔPMI (% change PMI/month) | −2.0 [−3.4, −1.3] | −0.15 [−0.65, 0.9] | <0.001* |
Months between CT scans | 9.4 [4.4, 12.3] | 7.9 [4.9, 10.7] | 0.74 |
Serum albumin at transplant (g/dL) | 2.6 [2.2, 2.9] | 3.1 [2.6, 3.4] | 0.03* |
Serum protein at transplant (g/dL) | 5.2 [3.8, 6.0] | 6.3 [5.4, 6.8] | 0.11 |
Data are presented as median [range] or n (%). *, P<0.05. PMI, psoas muscle index; VHW, very high wasting; LW, low wasting; Txp, transplant; MELD, Model for End-Stage Liver Disease; LT, liver transplantation; GFR, glomerular filtration rate; NASH, non-alcoholic steatohepatitis; HCC, hepatocellular carcinoma; BMI, body mass index; ΔPMI, change in PMI; CT, computed tomography.
Table 2 summarizes post-operative outcomes in this subset analysis. There were no significant differences in rates of early allograft dysfunction, primary nonfunction, or early post-operative complications, as characterized according to the Clavien-Dindo classification scheme. Patients in both groups also had similar durations of hospitalization and intensive care unit (ICU) admission. As mentioned above, 1-year patient and graft survival rates in VHW patients were significantly reduced.
Table 2
Variables | Wasting group | P value | |
---|---|---|---|
VHW (n=15) | LW (n=46) | ||
Peak serum ALT (U/L) | 479 [167, 867] | 457 [236, 856] | 0.73 |
Peak serum AST (U/L) | 563 [254, 1,021] | 729 [358, 1,463] | 0.37 |
Early allograft dysfunction | 1 (6.7) | 10 (21.7) | 0.19 |
Primary nonfunction | 0 | 0 | >0.99 |
Acute kidney injury | 6 (40.0) | 20 (43.5) | 0.81 |
Clavien-Dindo complication grade | 0.34 | ||
Grade 1 | 3 (20.0) | 10 (21.7) | |
Grade 2 | 1 (6.7) | 8 (17.4) | |
Grade 3 | 4 (26.7) | 6 (13.0) | |
Grade 4 | 4 (26.7) | 7 (15.2) | |
Grade 5 | 3 (20.0) | 2 (4.3) | |
Hospitalization duration (days) | 9 [7, 13] | 9.5 [7, 15.3] | 0.81 |
ICU duration (days) | 3 [2, 9] | 3 [2, 5.8] | 0.98 |
Days on ventilator | 1 [0, 2] | 1 [0, 2] | 0.78 |
Reintubation | 2 (13.3) | 2 (4.3) | 0.22 |
Tracheostomy | 1 (6.7) | 2 (4.3) | 0.72 |
90-day readmission | 7 (46.7) | 22 (47.8) | 0.94 |
Discharge disposition | n=12 | n=43 | 0.28 |
Home | 7 (58.3) | 32 (74.4) | |
Rehab | 5 (41.7) | 11 (25.6) | |
1-year graft survival (%) | 73.3 | 93.5 | 0.03* |
1-year patient survival (%) | 73.3 | 93.5 | 0.03* |
Data are presented as median [range], n (%), or percentage. *, P<0.05. PMI, psoas muscle index; VHW, very high wasting; LW, low wasting; ALT, alanine aminotransferase; AST, aspartate aminotransferase; ICU, intensive care unit.
Serum metabolomic analysis—median ΔPMI cutoff
Serum metabolomics analysis quantified 43 metabolites. Our initial analysis compared metabolite profiles between HWmed and LWmed groups, as defined by the median ΔPMI cutoff. ANOVA revealed that 12 metabolites demonstrated statistically different levels between at least two groups. With the application of Tukey post-hoc testing, it was revealed that all 12 were different between the LWmed and LKD groups and that seven were significantly different between the HWmed and LKD groups. No significant differences were observed between the HWmed and LWmed group. A table containing the fold changes and significance values between each of the groups is presented in Table S2. The significant differences are presented in the volcano plot in Figure 2.
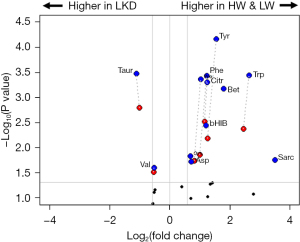
Perturbations to serum amino acids
The metabolomics platform included measurements for 17 of the canonical, proteogenic amino acids. Of these, six showed a significant difference in at least one of the liver disease groups. Proline was significantly increased in both the LWmed and HWmed groups as compared to healthy controls. Aspartate was increased only in the LWmed group compared to the LKD group. The three AAAs—phenylalanine, tryptophan, and tyrosine—demonstrated significant increases in both liver disease groups compared with the LKD group. The only amino acid that showed a reduction in the liver disease group was the BCAA valine. Consistent with this finding, there was also a trend towards reduction noted for leucine (LWmed vs. LKD: P=0.07; HWmed vs. LKD: P=0.08). The most significantly downregulated metabolite was taurine with a more than two-fold reduction in both the HWmed and LWmed groups as compared to LKD.
Perturbations to one-carbon metabolism
Several metabolites associated with one-carbon metabolism showed distinct elevations in the liver disease groups. One-carbon metabolism involves the folate-mediated movement of single carbon units i.e., methyl groups to facilitate a range of metabolic processes. This overall pathway includes the folate cycle, methionine salvage, and purine biosynthesis. Sarcosine showed the largest increase of any of these metabolites with a three-fold increase in the LWmed group as compared to healthy controls (P=0.001). There was also a nearly two-fold increase in the sarcosine levels in the HWmed group compared to LKD, however this difference was not statistically significant (P=0.06). Interestingly, the difference in sarcosine levels between HWmed and LWmed patients approached statistical significance (P=0.058). Betaine levels were significantly increased in HWmed and LWmed patients as compared to LKD. The amino acids tryptophan and methionine are also involved in the one-carbon metabolism pathway. Significant differences were observed between both liver disease groups and the LKDs for tryptophan and methionine.
Changes in serum lipoprotein profiles
There were significant decreases in seven of the lipoprotein particle concentrations in patients with liver disease compared to healthy controls. Additionally, the concentrations of total triglycerides and apolipoprotein (Apo)A1 were decreased as well. These results are shown in Figure 3 with raw data supplied in Table S3. Only the concentration of the very large triglyceride rich particles showed a difference between the HWmed and LWmed groups. The other lipoprotein particle changes all involved decreases in high-density lipoprotein (HDL) particles as compared to healthy controls. Consistent with this was a significant reduction in ApoA1, the major protein component of HDL particles.
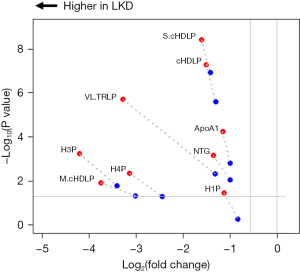
Serum metabolomic analysis—subgroup analysis (ΔPMI <−1%)
As we identified significantly worse 1-year survival in VHW patients, we performed an additional analysis of the metabolomics data including a separate group for the VWH. In this analysis, the initial HW group was separated into VHW (n=6) and HW (n=9). Table S4 shows the P values of all of the inter-group comparisons in this new analysis. The list of altered metabolites was nearly identical to the original analysis with the inclusion of one extra metabolite, a-hydroxyisovalerate (aHIV). As shown in Table S2, the P value for aHIV in the first analysis (comparing HWmed and LWmed) was 0.0511 and thus very nearly significant.
Discussion
The aim of this study was two-fold: (I) to establish the clinical relevance that the rate of muscle loss has on post-LT outcomes and (II) to identify patterns in small molecule metabolism unique to patients with rapidly progressive sarcopenia. While multiple studies have demonstrated the negative impact that sarcopenia has on post-LT outcomes (5-8), few have examined the rate at which this muscle loss develops. Single slice, axial CT imaging has been validated as an accurate measure of total body composition, and given the ubiquity of the technology, is an attractive tool for measurement of changes in body composition over time (15-17). Here we demonstrate that patients with more rapid muscle wasting (i.e., a decrease in PMI of >1%/month) had significantly worse 1-year patient and graft survival. Hanai et al. examine the relationship between the rate of muscle loss and mortality in cirrhotic patients (18). However, in this study, patients had a median percent change in PMI of −2.2%/year, and no outcomes after LT were considered. Our present study examines a population of patients with much more rapid muscle wasting (median ΔPMI in HW group =−2%/month), and specifically analyzes outcomes following LT. Our findings suggest that dynamic assessment of sarcopenia can identify a high-risk subset of patients who may need prioritization or careful consideration in terms of liver transplant candidacy to avoid futility.
Interestingly, there were no differences in early post-operative complications that might explain this difference in mortality between VHW and LW in our study. This suggests that the detrimental effects of rapid muscle wasting remain relevant even if patients progress through an uneventful perioperative course. Previous studies into the impact of sarcopenia on post-LT survival outcomes demonstrate that short- (1-year) and long-term (3-year) patient survival is inferior in patients with pre-LT sarcopenia (5). Additionally, pre-operative sarcopenia has been found to persist in the majority of post-LT patients, further supporting this observation (22,23). These studies used static measures of sarcopenia; long-term follow-up in our cohort can identify if similar trends exist in rapid wasters. It should be noted that our overall 1-year survival rate for the entire study population was 89% which is fairly in-line with published outcomes in the United States according to the most recent OPTN annual report (92.8% 1-year survival) (24). This 1-year survival rate improved to 93.5% when VHW patients were removed from the analysis.
The second part of the study focused on the serum metabolite profiles of patients with varying rates of muscle loss. Our goal was to search for metabolic perturbations that are associated with accelerated muscle wasting. A number of metabolomics analyses have been carried out on liver diseases and have identified specific metabolic perturbations associated with non-alcoholic fatty liver disease (NAFLD), cirrhosis, non-alcoholic steatohepatitis (NASH), etc. (25,26). These metabolic profiles have been utilized as a noninvasive screening tool for liver disease such as NASH and hepatic fibrosis (25). In this study we expected that some of these same metabolic perturbations would be observed in the liver disease subjects. Our hypothesis was that rate of muscle wasting in liver disease patients with sarcopenia would also influence the metabolome and that some of these perturbations would be detectable in addition to changes induced by liver disease. Such distinct changes could potentially serve as markers for patients who are at high risk from their sarcopenia for poor outcomes following LT. In our study, we did not identify specific changes in metabolites between HW and LW patients. However, we did find that, while metabolic perturbations generally appeared similar to those found in chronic liver disease, some distinct differences were also identified. These metabolic profiles, discussed ahead, indicated that the muscle metabolic status may indeed be influencing the circulating metabolome, and may suggest therapeutic interventions based on metabolic or nutritional supplementation.
A well-documented metabolic characteristic of liver cirrhosis is the decrease in BCAAs and an accompanying increase in AAAs (27,28). The reduction in BCAAs occurs primarily in the skeletal muscle, where these amino acids are utilized in reactions that form glutamate from a-ketoglutarate. The latter is a critical metabolite in the detoxification of ammonia that occurs with liver disease (29). The observed increase in AAA increase is due to the decreased ability of the diseased liver to metabolize these amino acids. A decrease in BCAAs is also observed in age-related muscle wasting (30-32). A potentially confounding factor to quantifying serum BCAAs is dietary intake. As mentioned earlier, cirrhotic patients often have reduced caloric intake. BCAA metabolism is very sensitive to changes in the amount and composition of food ingested, which is highly variable in both healthy and diseased states. Brief periods of starvation can acutely increase the circulating concentrations of BCAA (33) as a result of increased proteolysis and reduced protein synthesis (34). This makes the assessment of a patient’s true BCAA status difficult to ascertain. Although recent clinical trials have shown some improvement in muscle mass with BCAA supplementation (35), the major body of evidence in this field remains inconclusive (36). This discrepancy suggests that careful patient selection may be necessary for BCAA supplementation to achieve clinical benefits.
The impact of liver disease on one-carbon metabolism has been well-established (37). The liver is a major site of one-carbon metabolism, which plays a critical role in the transfer of one-carbon units through the folate cycle for remethylation of homocysteine. The metabolite betaine acts as a methyl donor in the remethylation of homocysteine to methionine. This reaction is catalyzed by the enzyme betaine hydroxymethyltransferase (BHMT). Teng et al. demonstrated that mice lacking BHMT have elevated liver betaine and homocysteine and rapidly develop HCC (38). The elevated betaine levels in the liver disease groups of our study suggest a similar dysregulation in BHMT. Conversely, in a study of sarcopenia in a cohort of community-dwelling Chinese adults, serum betaine levels were inversely correlated with low lean muscle mass (39). In our study, the betaine levels in the HW group were not different from the LKD. It is possible that the expected increase in betaine from the patient’s liver disease is offset in the HW group by a reduction in muscle mass.
Sarcosine also plays a role in the methionine remethylation pathway through the conversion of S-adenosylmethionine to S-adenosylhomocysteine. This reaction is catalyzed by the enzyme glycine-N-methyltransferase (GNMT) which also converts glycine to sarcosine. This significant elevation in sarcosine in the LW group may be related to a specific alteration to GNMT activity or may be the result of an upstream perturbation in BHMT. Interestingly, there was only a trending increase in the methionine levels in the LW group. As with betaine, the sarcosine levels are significantly increased in the LW group, but not in the HW group. Furthermore, sarcosine levels are very close to being significantly different between HW and LW. Currently, the literature on sarcosine and sarcopenia is rather sparse. A study of circulating amino acids in older persons with physical frailty found an increase in circulating sarcosine associated with physical frailty and sarcopenia (40). A reduction in circulating sarcosine was associated with aging in both rodents and humans (41). Although there were no significant differences in the ages of the HW and LW groups, it is interesting to consider that the HW group may have distinct metabolic features of aging that contribute to the observed sarcosine levels.
Taurine is a non-proteogenic amino acid and is the most abundant free amino acid in skeletal muscle. It is involved in a wide range of functions including the response to oxidative stress and inflammation (42). Taurine levels are maintained in the liver by both endogenous biosynthesis and exogenous transport, and are typically decreased in liver diseases (43). A significant decrease in taurine levels is also observed in age-associated sarcopenia (44,45). Our findings are consistent with this knowledge. It is interesting to note that therapeutic supplementation with taurine has been studied to treat metabolic imbalances in both liver diseases and sarcopenia (46,47) and thus taurine supplementation may be beneficial in liver disease patients irrespective of the degree and rate of sarcopenia.
The liver plays a critical role in lipoprotein metabolism, contributing functions such as lipid synthesis and transport. Patients with cirrhosis often have several defects in lipid metabolism, such as hypocholesterolemia in association with reduced levels of HDL cholesterol (HDL-C), low-density lipoprotein cholesterol (LDL-C), and ApoA1 (48). HDL levels have been shown to decrease with increasing severity of liver disease (40,49). Our results showed a consistent decrease in HDLs as measured by the HDL particle numbers (HDL-P). A similar reduction in HDLs has been observed in a study of sarcopenic patient with chronic obstructive pulmonary disease (COPD) with HDL levels being an independent predictor of sarcopenia in both controls and COPD patients (50).
It should be noted that this study was performed at a single institution and includes a relatively small sample size. Although our program performed over 1,500 transplants during the study period, only 61 patients qualified. This was mainly a result of the fact that the average waitlist time for a liver transplant at our center is less than 4 months, which means that many patients did not have the requisite >4-month gap between successive CT scans. Due to the scope of this study, a number of social determinants of health (i.e., socioeconomic class, insurance status, access to nutritious foods, etc.) were not accounted for. These may indirectly influence the progression of muscle loss in future transplant recipients and attempts to control for these factors should be made in future studies.
Conclusions
The rate of progression of sarcopenia in ESLD patients awaiting transplant is a strong prognostic indicator of worse post-transplant survival. Metabolite profiles did not differ between HW and LW patients, but the overall patterns most closely resembled derangements associated with chronic liver disease. While we were unable to identify a “high risk” metabolic profile, metabolic perturbations identified herein suggest that nutritional interventions aimed at maintaining muscle mass may benefit liver transplant patients irrespective of the rate of sarcopenic progression.
Acknowledgments
We would like to thank Dr. Peter Thompson at the Molecular Education, Technology and Research Innovation Center (METRIC) at NC State University for collection of the NMR data.
Funding: None.
Footnote
Reporting Checklist: The authors have completed the STROBE reporting checklist. Available at https://hbsn.amegroups.com/article/view/10.21037/hbsn-23-645/rc
Data Sharing Statement: Available at https://hbsn.amegroups.com/article/view/10.21037/hbsn-23-645/dss
Peer Review File: Available at https://hbsn.amegroups.com/article/view/10.21037/hbsn-23-645/prf
Conflicts of Interest: All authors have completed the ICMJE uniform disclosure form (available at https://hbsn.amegroups.com/article/view/10.21037/hbsn-23-645/coif). The authors have no conflicts of interest to declare.
Ethical Statement: The authors are accountable for all aspects of the work in ensuring that questions related to the accuracy or integrity of any part of the work are appropriately investigated and resolved. All research was conducted in accordance with both the Declarations of Helsinki (as revised in 2013) and Istanbul. Analysis of patient data for this study was reviewed and approved by the Institutional Review Board of the Indiana University School of Medicine (IRB #1305011413) and individual consent for this retrospective analysis was waived.
Open Access Statement: This is an Open Access article distributed in accordance with the Creative Commons Attribution-NonCommercial-NoDerivs 4.0 International License (CC BY-NC-ND 4.0), which permits the non-commercial replication and distribution of the article with the strict proviso that no changes or edits are made and the original work is properly cited (including links to both the formal publication through the relevant DOI and the license). See: https://creativecommons.org/licenses/by-nc-nd/4.0/.
References
- Fuggle N, Shaw S, Dennison E, et al. Sarcopenia. Best Pract Res Clin Rheumatol 2017;31:218-42. [Crossref] [PubMed]
- Scaglione S, Kliethermes S, Cao G, et al. The Epidemiology of Cirrhosis in the United States: A Population-based Study. J Clin Gastroenterol 2015;49:690-6. [Crossref] [PubMed]
- Amaral TF, Matos LC, Tavares MM, et al. The economic impact of disease-related malnutrition at hospital admission. Clin Nutr 2007;26:778-84. [Crossref] [PubMed]
- Hanai T, Shiraki M, Nishimura K, et al. Sarcopenia impairs prognosis of patients with liver cirrhosis. Nutrition 2015;31:193-9. [Crossref] [PubMed]
- Englesbe MJ, Patel SP, He K, et al. Sarcopenia and mortality after liver transplantation. J Am Coll Surg 2010;211:271-8. [Crossref] [PubMed]
- Montano-Loza AJ, Meza-Junco J, Baracos VE, et al. Severe muscle depletion predicts postoperative length of stay but is not associated with survival after liver transplantation. Liver Transpl 2014;20:640-8. [Crossref] [PubMed]
- Tandon P, Ney M, Irwin I, et al. Severe muscle depletion in patients on the liver transplant wait list: its prevalence and independent prognostic value. Liver Transpl 2012;18:1209-16. [Crossref] [PubMed]
- Montano-Loza AJ, Meza-Junco J, Prado CM, et al. Muscle wasting is associated with mortality in patients with cirrhosis. Clin Gastroenterol Hepatol 2012;10:166-73, 173.e1.
- Sinclair M, Gow PJ, Grossmann M, et al. Review article: sarcopenia in cirrhosis--aetiology, implications and potential therapeutic interventions. Aliment Pharmacol Ther 2016;43:765-77. [Crossref] [PubMed]
- Dichi JB, Dichi I, Maio R, et al. Whole-body protein turnover in malnourished patients with child class B and C cirrhosis on diets low to high in protein energy. Nutrition 2001;17:239-42. [Crossref] [PubMed]
- Dam G, Sørensen M, Buhl M, et al. Muscle metabolism and whole blood amino acid profile in patients with liver disease. Scand J Clin Lab Invest 2015;75:674-80. [PubMed]
- Soeters PB, Fischer JE. Insulin, glucagon, aminoacid imbalance, and hepatic encephalopathy. Lancet 1976;2:880-2. [Crossref] [PubMed]
- Lin SY, Chen WY, Lee FY, et al. Activation of ubiquitin-proteasome pathway is involved in skeletal muscle wasting in a rat model with biliary cirrhosis: potential role of TNF-alpha. Am J Physiol Endocrinol Metab 2005;288:E493-501. [Crossref] [PubMed]
- Shaw KA, Srikanth VK, Fryer JL, et al. Dual energy X-ray absorptiometry body composition and aging in a population-based older cohort. Int J Obes (Lond) 2007;31:279-84. [Crossref] [PubMed]
- Shen W, Punyanitya M, Wang Z, et al. Total body skeletal muscle and adipose tissue volumes: estimation from a single abdominal cross-sectional image. J Appl Physiol (1985) 2004;97:2333-8. [Crossref] [PubMed]
- Giusto M, Lattanzi B, Albanese C, et al. Sarcopenia in liver cirrhosis: the role of computed tomography scan for the assessment of muscle mass compared with dual-energy X-ray absorptiometry and anthropometry. Eur J Gastroenterol Hepatol 2015;27:328-34. [Crossref] [PubMed]
- Bush WJ, Davis JP, Maluccio MA, et al. Computed Tomography Measures of Nutrition in Patients With End-Stage Liver Disease Provide a Novel Approach to Characterize Deficits. Transplant Proc 2018;50:3501-7. [Crossref] [PubMed]
- Hanai T, Shiraki M, Ohnishi S, et al. Rapid skeletal muscle wasting predicts worse survival in patients with liver cirrhosis. Hepatol Res 2016;46:743-51. [Crossref] [PubMed]
- Braunschweig CA, Sheean PM, Peterson SJ, et al. Exploitation of diagnostic computed tomography scans to assess the impact of nutrition support on body composition changes in respiratory failure patients. JPEN J Parenter Enteral Nutr 2014;38:880-5. [Crossref] [PubMed]
- Jeyarajah EJ, Cromwell WC, Otvos JD. Lipoprotein particle analysis by nuclear magnetic resonance spectroscopy. Clin Lab Med 2006;26:847-70. [Crossref] [PubMed]
- Choudhury R, Beezley J, Davis B, et al. Viime: Visualization and Integration of Metabolomics Experiments. J Open Source Softw 2020;5:2410. [Crossref] [PubMed]
- Lai JC, Segev DL, McCulloch CE, et al. Physical frailty after liver transplantation. Am J Transplant 2018;18:1986-94. [Crossref] [PubMed]
- Bhanji RA, Takahashi N, Moynagh MR, et al. The evolution and impact of sarcopenia pre- and post-liver transplantation. Aliment Pharmacol Ther 2019;49:807-13. [Crossref] [PubMed]
- Kwong AJ, Ebel NH, Kim WR, et al. OPTN/SRTR 2021 Annual Data Report: Liver. Am J Transplant 2023;23:S178-263. [Crossref] [PubMed]
- Caussy C, Ajmera VH, Puri P, et al. Serum metabolites detect the presence of advanced fibrosis in derivation and validation cohorts of patients with non-alcoholic fatty liver disease. Gut 2019;68:1884-92. [Crossref] [PubMed]
- Wang X, Rao B, Wang H, et al. Serum metabolome alterations in patients with early nonalcoholic fatty liver disease. Biosci Rep 2022;42:BSR20220319. [Crossref] [PubMed]
- Dasarathy S, Hatzoglou M. Hyperammonemia and proteostasis in cirrhosis. Curr Opin Clin Nutr Metab Care 2018;21:30-6. [Crossref] [PubMed]
- Dejong CH, van de Poll MC, Soeters PB, et al. Aromatic amino acid metabolism during liver failure. J Nutr 2007;137:1579S-1585S; discussion 1597S-1598S. [Crossref] [PubMed]
- Holecek M, Kandar R, Sispera L, et al. Acute hyperammonemia activates branched-chain amino acid catabolism and decreases their extracellular concentrations: different sensitivity of red and white muscle. Amino Acids 2011;40:575-84. [Crossref] [PubMed]
- Lu Y, Karagounis LG, Ng TP, et al. Systemic and Metabolic Signature of Sarcopenia in Community-Dwelling Older Adults. J Gerontol A Biol Sci Med Sci 2020;75:309-17. [PubMed]
- Meng L, Yang R, Wang D, et al. Specific lysophosphatidylcholine and acylcarnitine related to sarcopenia and its components in older men. BMC Geriatr 2022;22:249. [Crossref] [PubMed]
- Yamada M, Kimura Y, Ishiyama D, et al. Plasma Amino Acid Concentrations Are Associated with Muscle Function in Older Japanese Women. J Nutr Health Aging 2018;22:819-23. [Crossref] [PubMed]
- Holeček M. Branched-chain amino acids in health and disease: metabolism, alterations in blood plasma, and as supplements. Nutr Metab (Lond) 2018;15:33. [Crossref] [PubMed]
- Holeček M, Mičuda S. Amino acid concentrations and protein metabolism of two types of rat skeletal muscle in postprandial state and after brief starvation. Physiol Res 2017;66:959-67. [Crossref] [PubMed]
- Siramolpiwat S, Limthanetkul N, Pornthisarn B, et al. Branched-chain amino acids supplementation improves liver frailty index in frail compensated cirrhotic patients: a randomized controlled trial. BMC Gastroenterol 2023;23:154. [Crossref] [PubMed]
- van Dijk AM, Bruins Slot AS, Portincasa P, et al. Systematic review with meta-analysis: Branched-chain amino acid supplementation in liver disease. Eur J Clin Invest 2023;53:e13909. [Crossref] [PubMed]
- da Silva RP, Eudy BJ, Deminice R. One-Carbon Metabolism in Fatty Liver Disease and Fibrosis: One-Carbon to Rule Them All. J Nutr 2020;150:994-1003. [Crossref] [PubMed]
- Teng YW, Mehedint MG, Garrow TA, et al. Deletion of betaine-homocysteine S-methyltransferase in mice perturbs choline and 1-carbon metabolism, resulting in fatty liver and hepatocellular carcinomas. J Biol Chem 2011;286:36258-67. [Crossref] [PubMed]
- Huang BX, Zhu YY, Tan XY, et al. Serum betaine is inversely associated with low lean mass mainly in men in a Chinese middle-aged and elderly community-dwelling population. Br J Nutr 2016;115:2181-8. [Crossref] [PubMed]
- Calvani R, Picca A, Marini F, et al. A Distinct Pattern of Circulating Amino Acids Characterizes Older Persons with Physical Frailty and Sarcopenia: Results from the BIOSPHERE Study. Nutrients 2018;10:1691. [Crossref] [PubMed]
- Walters RO, Arias E, Diaz A, et al. Sarcosine Is Uniquely Modulated by Aging and Dietary Restriction in Rodents and Humans. Cell Rep 2018;25:663-676.e6. [Crossref] [PubMed]
- Lambert IH, Kristensen DM, Holm JB, et al. Physiological role of taurine--from organism to organelle. Acta Physiol (Oxf) 2015;213:191-212. [Crossref] [PubMed]
- Miyazaki T, Matsuzaki Y. Taurine and liver diseases: a focus on the heterogeneous protective properties of taurine. Amino Acids 2014;46:101-10. [Crossref] [PubMed]
- Miyamoto K, Hirayama A, Sato Y, et al. A Metabolomic Profile Predictive of New Osteoporosis or Sarcopenia Development. Metabolites 2021;11:278. [Crossref] [PubMed]
- Yeung SSY, Zhu ZLY, Kwok T, et al. Serum Amino Acids Patterns and 4-Year Sarcopenia Risk in Community-Dwelling Chinese Older Adults. Gerontology 2022;68:736-45. [Crossref] [PubMed]
- Barbiera A, Sorrentino S, Lepore E, et al. Taurine Attenuates Catabolic Processes Related to the Onset of Sarcopenia. Int J Mol Sci 2020;21:8865. [Crossref] [PubMed]
- Zhang Z, Liu D, Yi B, et al. Taurine supplementation reduces oxidative stress and protects the liver in an iron-overload murine model. Mol Med Rep 2014;10:2255-62. [Crossref] [PubMed]
- Rubiés-Prat J, Masdeu S, Nubiola AR, et al. High-density lipoprotein cholesterol and phospholipids, and apoprotein A in serum of patients with liver disease. Clin Chem 1982;28:525-7. [Crossref] [PubMed]
- Poynard T, Abella A, Pignon JP, et al. Apolipoprotein AI and alcoholic liver disease. Hepatology 1986;6:1391-5. [Crossref] [PubMed]
- Abu-Libdeh W, Khrais J, Suwan L, et al. Relation of Plasma High-Density Lipoproteins-Cholesterol with Sarcopenia in Patients with Chronic Obstructive Pulmonary. Indiana J Respir Care 2022;11:327-32. [Crossref]