Liver venous deprivation (LVD) before extended hepatectomy: a French multicentric retrospective cohort
Highlight box
Key findings
• Liver venous deprivation (LVD) is a safe, reproducible technique that is effective in a large cohort of patients requiring major hepatectomy with insufficient future liver remnant.
What is known and what is new?
• LVD has been shown to be superior to portal vein embolization in terms of hypertrophy in small retrospective studies.
• This multicentric cohort is the largest described and confirms these results in terms of feasibility, reproducibility and efficacy.
What is the implication, and what should change now?
• The indication and technique of LVD will require more standardization in the future in order to further improve its results.
Introduction
Hepatic resection surgery remains the gold standard for curative management of hepatic malignant tumors, both primary and secondary. After major hepatic resection of large, multiple, and/or bilobar lesions, there is a risk of post-hepatectomy liver failure (PHLF), especially in patients with underlying cirrhosis, steatosis, or those who have received multiple cycles of chemotherapy (1,2). PHLF remains the prime cause of postoperative mortality after major hepatic resection (3). The risk of clinically relevant PHLF in patients with future liver remnants (FLRs) <20% (or an FLR to bodyweight ratio <0.5) (4) of a healthy liver or <30–35% (or an FLR to bodyweight ratio <0.5–0.8) of diseased liver is up to 40% (5).
Prevention of PHLF has been a major surgical challenge over the last three decades. Parenchyma-sparing has been developed in those undergoing multiple or single resections (6,7) and preoperative venous embolization has been employed to induce FLR volume hypertrophy.
Portal vein embolization (PVE) has become the standard method for induction of FLR hypertrophy before major hepatectomy ever since the first description thereof by Makuuchi et al. in 1984 (8). Despite the demonstrated efficiency and safety of PVE (9-11), 20% to 30% of patients drop out before surgery mainly because of tumor progression associated with insufficient FLR hypertrophy, or procedural failure (10,12). Such tumor progression is definitely attributable to the delay between PVE and surgery (4–8 weeks). The associating liver partition and portal vein ligation for staged hepatectomy (ALPPS) method was described in 2012 (13), and is supposed to induce FLR hypertrophy within 7 days (14). However, this procedure is associated with significant morbidity and mortality, rendering generalization difficult (15). In 2009, Hwang et al. (16) described sequential embolization of the portal and then the hepatic vein embolization (HVE). This is an improved form of liver venous deprivation (LVD) (17-20) in one procedure that offers more rapid FLR hypertrophy before hepatic resection than does PVE. This procedure is being developed further for patients at risk of PVE failure and is currently used in several specialist centers. The aim of this register was to explore the characteristics of LVD in terms of feasibility, efficacy, and safety in a large, retrospective, French multicentric cohort. We present this article in accordance with the STROCSS reporting checklist (available at https://hbsn.amegroups.com/article/view/10.21037/hbsn-24-315/rc).
Methods
Patient selection
This is a retrospective multicentric study of patients eligible for right hepatectomy (extended or not to segments I and IV), to treat hepatic malignancies (primary or metastatic), who lacked sufficient FLR volumes and thus underwent LVD procedures. Patients from seven University Hospital Centers of France that employ LVD procedures were included.
Liver volumes were measured using Myrian Software® (Intrasense, Montpelier, France) or Synapse 3D® (Fujifilm, Tokyo, Japan) to evaluate computed tomography (CT) scans taken before each procedure by trained radiologists or surgeons. Patients underwent surgery if adequate FLR growth and no oncological progression were evident on the control CT scans.
FLR volumes were measured in milliliters, and the percentage of remaining liver (%FLR) compared to the total liver volume (TLV). Also, the percentage of remaining liver divided by patient bodyweight (%FLR/BW) was calculated.
The indications for LVD were an FLR <25% [or an FLR/weight (kg) ≤0.5] for a healthy liver and an FLR <30% [or an FLR/weight (kg) ≤0.8] for a diseased liver.
A diseased liver was defined as a liver at increased risk of PHLF because of any of obesity [body mass index (BMI) >30 kg/m2], diabetes, steatosis, 3–6 months of preoperative chemotherapy (including irinotecan or oxaliplatin) that increased the risk of steatosis, sinusoidal obstruction syndrome (SOS) (a blue liver), liver fibrosis, and/or signs of portal hypertension on imaging or biological evaluation (liver aspect, splenomegaly, thrombocythemia of the portosystemic collaterals). Patients with liver cirrhosis were not included in this study.
For patients with bilobar colorectal metastatic disease, we used a two-step approach: clearance of the remaining liver followed by LVD as soon as possible, and right hepatectomy after 4 weeks reassessment. For patients with biliary tract carcinoma with jaundice, biliary drainage was performed before LVD or at the same time during a combined procedure.
The study was conducted in accordance with the Declaration of Helsinki (as revised in 2013). Inform consent was obtained at the surgical consultation or during hospital stay. This research was retrospectively registered and validated by Bordeaux University Hospital ethical committee (No. CER-BDX 2024-202).
LVD procedure
LVD procedures were performed by trained radiologists with patients under general anesthesia.
The right portal vein (PV) was percutaneously catheterized under ultrasound guidance and complete right portography was then performed. PVE proceeded by injecting in distal portal branches a mixture of iodized oil (lipiodol) and n-butyl-cyanoacrylate. None of the patients in the series had segment IV branch embolization and we specified this in the manuscript. The distal regions of the right hepatic vein (RHV) and, in some patients, the median hepatic vein (MHV), were catheterized to insert an Amplatzer® vascular plug. Hepatic vein approach could be transhepatic or transjugular, depending on the practice of each center. A transjugular approach is usually preferred for biliary tract cancers with biliary tract dilatation in order to avoid bile leakage by transhepatic approach. Contrast medium was injected to identify distal branches of the RHV, which were then selectively embolized along the catheter path using the same lipiodol and n-butyl-cyanoacrylate mixture (Figure 1). Venous collaterals found by the radiologist could be selectively embolised during the procedure.
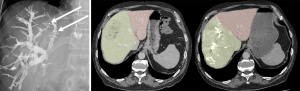
LVD safety and efficacy
Blood tests were performed 1 day after each procedure to monitor liver function [aspartate aminotransferase, alanine aminotransferase, and bilirubin levels; and the prothrombin time (PT)]; the hemoglobin level; and inflammatory syndrome status (white blood cell count and the C-reactive protein level).
Post-procedural pain was assessed, as was the need for opioids.
Morbidity and mortality were evaluated at 30 days using the Clavien-Dindo classification. Minor complications, defined as grade I or II, were adverse events with no or minimal impact on the postoperative course. Severe complications, defined as grade III or IV, were considered markers of major morbidity.
Control liver volumetry was performed 3–4 weeks after each procedure; a second CT scan was obtained to determine FLR growth. The volumetric parameters described above (FLR; %FLR; %FLR/BW) were again measured; the FLR percentage growth was the ratio of the %FLR after the procedure to the %FLR before the procedure. The kinetic growth rate (KGR) was the degree of hypertrophy divided by the number of weeks between LVD and volumetric reassessment. The degree of hypertrophy was thus the difference between the FLR% values of the initial assessment and the reassessment.
Surgery
Surgery was performed in patients with sufficient hypertrophy, defined as FLR >30% of the normal liver [and/or an FLR/weight (kg) ratio >0.5], FLR >40% of the underlying diseased liver [and/or an FLR/weight (kg) ratio >0.8]. The type of liver surgery was decided by the surgical staff who examined the liver volumetric CT scans and liver magnetic resonance imaging (MRI) results. Perioperative hepatic ultrasound was used to monitor surgical progress by the number of liver tumors and their relationships with vasculo-biliary structures. All procedures in this study were right hepatectomies that could be extended to segments I and IV and were performed by expert hepatobiliary surgeons. Commencing with extrahepatic division, and ligation of the pedicular vessels and hepatic vein, liver resection proceeded by employing ultrasonic dissection, electrocoagulation, clips, and ligations. Lymph node dissection was guided by the oncological recommendations and the perioperative findings. All operative decisions were taken in a multidisciplinary meeting, and major hepatectomy was decided only if there was no parenchyma sparing alternative.
Postoperative outcomes
Blood tests were regularly scheduled postoperatively to monitor any biological sign of bleeding, inflammatory syndrome, and PHLF according to International Study Group of Liver Surgery (ISGLS) definition. Liver biological function was assessed by measuring the international normalized ratio (INR), the PT, and the bilirubin level. The definition of PHLF was that of the consensus agreed to by the ISGLS (2), thus, an increased INR and/or serum bilirubin level and a decreased PT from the 5th postoperative day; there are three PHLF severity grades. Grade A features only abnormal liver biological parameters with no consequences for the clinical management of the patient; Grade B is associated with a change in clinical management but no invasive treatment; Grade C is associated with invasive treatment and/or a need for intensive care. Postoperative complications were recorded at 30 and 90 days and were scored using the Clavien-Dindo classification as outlined above. Postoperative mortality was defined as death from any cause within 90 days of the procedure or during the hospital stay. That stay was calculated from the first day to discharge or death.
Statistical analysis
Quantitative data are expressed as means ± standard deviations or medians with ranges. Qualitative data are expressed as frequencies with percentages. All data are available on reasonable request. For comparison tests between groups, differences were determined using a χ2 test. A P value of ≤0.05 was considered statistically significant.
Results
Patient characteristics
Between January 2016 and November 2023, 192 patients underwent LVD in seven French University Hospital Centers, all of which commonly use LVD to increase FLR volume before major liver resection. Patient characteristics and initial volumetric data are listed in Table 1. Colorectal liver metastasis (CRLM) was the first indication for LVD (127 patients, 66.1%), of whom 66 (51.9%) had initial bilobar metastases and 48 (37.8%) underwent left liver cleaning via wedge resection and radiofrequencies several weeks before right major two-stage hepatectomy. Median number of metastases in the FLR was 2 (1-8), 5 patients had at least one radiofrequency procedure concomitant with wedge resections. For these 48 patients, LVD was performed during the same first hospitalization. The vast majority of patients with CRLM (85%) received at least 3 months of chemotherapy before LVD. Details for preoperative systemic treatments for CRLM patients are available in Table S1. For 11 (50%) patients with cholangiocarcinomas (CCAs) (11/22), preoperative biliary drainage were performed before or at the same time as LVD.
Table 1
Baseline characteristics | Values |
---|---|
Sex | |
Male | 114 [59] |
Female | 78 [41] |
Age (years) | 64±10 |
BMI (kg/m2) | 25.2±4.3 |
ASA grade | |
1 | 28 [14.6] |
2 | 128 [66.7] |
3 | 36 [18.7] |
Liver tumor | |
CRLM | 127 [66.1] |
HCC | 29 [15.1] |
NET | 3 [1.6] |
CCA | 22 [11.5] |
Others | 11 [5.7] |
TLV (mm3) | 1,566±374 |
FLR (mm3) | 391±134 |
%FLR | 25.2±7.5 |
FLR%/BW ratio | 0.54±0.18 |
Data are presented as n [%] and mean ± standard deviation. BMI, body mass index; ASA, American Society of Anesthesiologists; CRLM, colorectal liver metastasis; HCC, hepatocellular carcinoma; NET, neuroendocrine tumor; CCA, cholangiocarcinoma; TLV, total liver volume; FLR, future liver remnant; BW, bodyweight.
Feasibility and safety of LVD
LVD was technically feasible for all patients; there was no procedural failure. None of the patients in the series had segment IV branch embolization. Significant complications (Clavien-Dindo II to IVA) were observed in 22 (11.4%) patients and severe complications (Clavien-Dindo ≥ IIIA) in 5 (2.6%). Two patients (1.04%) developed liver failure with ascitic decompensation that required prolonged hospitalization and one could not undergo surgery because of refractory ascites. One patient who developed a biliary tract wound during the procedure was treated via radiological drainage. One patient experienced thoracic migration of the hepatic vein plug into the vena cava that required surgical removal via a thoracic approach (Figure 2). The most common complications were pain (21 patients, 10.9%) and/or fever (15 patients, 7.8%) but with no clinical, biological, or CT issues, and with a favorable evolution. The median length of hospital stay was 2 days (range, 1–25 days) but hospitalization could be prolonged for patients with bilobar metastases because left liver cleaning was performed during the same hospital stay (before LVD). The feasibility and safety of LVD are described in Table 2.
Table 2
Values | |
---|---|
LVD | |
RHV | 172 (89.6) |
RHV + MHV | 20 (10.4) |
Clavien-Dindo | |
II | 22 (11.4) |
Pain | 21 (10.9) |
Fever | 15 (7.8) |
Liver abscess | 1 (0.52) |
Left embolism | 4 (2.1) |
Hemoperitoneum | 2 (1.04) |
IIIA | 0 |
IIIB | 2 (1.04) |
Biliary wound | 1 (0.52) |
Sepsis | 1 (0.52) |
IV | 3 (1.56) |
Vena cava migration | 1 (0.52) |
Liver failure | 2 (1.04) |
Length of hospital stay (days) | 2 [1–25] |
Data are presented as n (%) and median [range]. LVD, liver venous deprivation; RHV, right hepatic vein; MHV, median hepatic vein.
Efficacy of LVD
The mean time to volumetric reassessment was 27±9.7 days; contrast-enhanced CT scans were reviewed by radiologists trained in liver volumetric measurements. The volumetric characteristics are described in Table 3. The FLR volume and %FLR increased by 61.7% and 59.2% respectively. The mean FLR%/BW ratio after LVD was 0.9 and the KGR was (6±5.1)% per week.
Table 3
Values | |
---|---|
Time to reassessment (days) | 27±9.7 |
TLV (mm3) | 1,637±389 |
RLV (mm3) | 623±188 |
Post LVD %FLR | 39±8 |
FLR%/BW | 0.9±0.31 |
FLR volume growth (%) | 61.7±48.49 |
KGR (%/week) | 6±5.1 |
Data are presented as mean ± standard deviation. LVD, liver venous deprivation; TLV, total liver volume; RLV, resected liver volume; FLR, future liver remnant; BW, bodyweight; KGR, kinetic growth rate.
Surgery
Thirty-one (16.2%) patients could not undergo major hepatic resection after LVD. Twenty-eight (14.6%) had tumor progression and developed intra-hepatic or metastatic disease progression between LVD and reassessment, including 20 patients treated for CRLM (20/28). The CRLM drop-out rate for oncological progression is therefore 15.7% (20/127).
Two patients (1.1%) exhibited insufficient FLR% growth at the 2-month reassessments, and 1 (0.5%) developed persistent liver failure after LVD. Of all patients, 57.1% (92/161) underwent conventional right hepatectomy (with or without extension to segment I) and 42.9% (69/161) segment IV-extended hepatectomy with or without extension to segment I. The surgical characteristics are listed in Table 4.
Table 4
Values | |
---|---|
Surgery | |
Yes | 161 (83.8) |
No | 31 (16.2) |
Time to surgery (days) | 40 [13–172] |
Procedure type | |
RH | 92 (57.1) |
ERH | 69 (42.9) |
Combined procedures | |
Left tumorectomies | 15 (9.3) |
Left RF | 7 (4.3) |
Data are presented as n (%) and median [range]. RH, right hepatectomy; ERH, extended right hepatectomy; RF, radiofrequency.
The percentage of severe postoperative complications (Clavien-Dindo ≥ IIIA) was 21.1% (34/161) including a postoperative mortality rate (Clavien-Dindo V) of 4.3% (7/161). Thirty-seven patients (22.9%) presented with a post-operative ascites flare-up, and 16 patients (9.9%) presented with a post-hepatectomy biliary leakage. The percentage of clinically significant PHLF (grade B or C) was 21.1% (34/161) including a grade C PHLF rate of 6.8% (11/161). The surgical complications are listed in Table 5. The characteristics of patients with clinically significant PHLF (grade B or C) and without clinically significant PHLF (patients without PHLF or of grade A) are described in Table 6. The proportion of CCAs was significantly higher in the PHLF B/C group compared to the other group (P=0.002); the former group also exhibited a lower FLR% on reassessment (P=0.02), and a higher rate of right hepatectomy that extended to segment IV (P=0.02). Five patients (3.1%) died within 90 days of surgery from grade C PHLF, 3 of them had CCA, 4 of them had an extended right hepatectomy. For these 5 patients, the initial FLR was 17.8%±3.8% and the re-evaluation FLR was 26%±5.5%.
Table 5
Values | |
---|---|
Clavien-Dindo grade | |
II | 41 (25.46) |
IIIA | 15 (9.3) |
IIIB | 8 (4.9) |
IV | 4 (2.5) |
PHLF | |
A | 9 (5.6) |
B | 23 (14.3) |
C | 11 (6.8) |
Mortality to 90 days | 7 (4.3) |
PHLF grade C | 5 (3.1) |
Portal thrombosis | 1 (0.6) |
Pneumopathy | 1 (0.6) |
Hospital stay (days) | 9 [3–55] |
Data are presented as n (%) and median [range]. PHLF, post-hepatectomy liver failure.
Table 6
PHLF B/C (n=34) | PHLF 0/A (n=127) | P value | |
---|---|---|---|
Age (years) | 65.7±8.4 | 64±10.2 | 0.48 |
BMI (kg/m2) | 25±4.9 | 25.2±4 | 0.52 |
Primary tumor | |||
CRLM | 18 (52.9) | 95 (74.8) | 0.07 |
HCC | 5 (14.7) | 18 (14.2) | 0.78 |
CCA | 10 (29.4) | 11 (8.6) | 0.002 |
Initial FLR% | 25.5±9.3 | 25.1±6 | 0.14 |
Reassessment FLR% | 37±10 | 39±8.6 | 0.02 |
Procedure type | 0.02 | ||
RH | 12 (35.3) | 78 (61.4) | |
ERH | 22 (64.7) | 49 (38.6) |
PHLF, post-hepatectomy liver failure; BMI, body mass index; CRLM, colorectal liver metastasis; HCC, hepatocellular carcinoma; CCA, cholangiocarcinoma; FLR, future liver remnant; RH, right hepatectomy; ERH, extended right hepatectomy.
Discussion
This national registry aimed to report the feasibility, safety, and efficacy of LVD in seven French University Hospital Centers. As the indications for LVD remain unclear, it is important to understand how and why this technique is currently used, and what the results are on a national scale. The primary objective of LVD is to increase the resectability of liver malignancies, especially in patients at risk of PVE failure.
The feasibility and reproducibility results were satisfactory; 100% of all LVDs were performed without technical failure at the seven different centers. The overall complication rate of 14% and the severe (Clavien-Dindo ≥ IIIA) complication rate of 2.6% following LVD procedure, are relatively low despite the one case of thoracic migration, which is thus a theoretical risk associated with the procedure (16,21), especially when the hepatic vein is catheterized transhepatically. This risk is supposed to be reduced when using a transjugular approach, enabling a plug that is 80–100% thicker than the hepatic vein to be deposited (22). However, in this particular case, the approach was transjugular with the insertion of a 20 mm vascular Amplatzer II plug in the RHV, and to date we have not been able to understand the mechanism of this complication. The rates of overall complications are similar to those of previous comparative retrospective studies (LVD vs. PVE) as are the rates of severe complications (Clavien-Dindo ≥ IIIA), which ranged from 0% to 3% depending on the series (17,18,22,23). The main cause of morbidity was post-procedure pain or fever with no clinical, biological, or CT findings and with spontaneously favorable evolution. Such symptoms are common after portal or arterial embolization, or chemoembolization, and are classically termed “post-embolization syndrome” (PES) (24). PES develops principally after arterial chemoembolization and includes a group of non-specific symptoms such as pain, fever, nausea, and vomiting.
PES occurs within the first 72 h after embolization of a solid organ and classically rapidly resolves after symptomatic treatment.
We observed an FLR% growth of 61.7% over 27 days. These results are consistent with those of previous studies: Chebaro et al. (25) observed an FLR% growth of 63% over 28 days; the figure of Laurent et al. (18) was 61.2% over 30 days. These results highlight the efficacy of LVD; the FLR% growth is nearly twice that after PVE (10,18,26). In this study, the KGR after LVD was (6±5.1)% per week; few KGR data after LVD are available. Indeed, several retrospective studies that measured the KGR after LVD reported very different results [KGR =(3.9–19)% per week] over different time intervals (13–41 days) (18,22,23,27,28). However, Shindoh et al. (29) found that a KGR <2% or >2% per week correlated with the PHLF risk after PVE (0% vs. 21% respectively, P<0.0001). These results must be interpreted with caution, however, as hypertrophy is non-linear, and the time before re-evaluation may affect the KGR. This interval must be identical if the KGRs of two groups are to be compared.
The drop-out rate of our study came to 16.2%, almost all of which (14.6%) was attributable to tumor progression. This is lower than that after PVE (20–30%) (10,11). Our resectability rate is comparable to those of smaller-volume retrospective comparative studies (LVD vs. PVE), with resection rates of 81–100% (18,22,23,28). This may reflect the fact that the time to liver resection is shorter after LVD than PVE (4–6 weeks) (30), reducing the risk of tumor progression during this period. However, we observed a median time to surgery of 40 days. This is surprisingly long, but may be explained by logistical limitations and a fear that the FLR% growth was insufficient before that time. The main volume gain occurs as early as day 7 post-procedure (17).
In a 2016 study, Guiu et al. demonstrated that liver resection on day 15 post-LVD was safe and feasible (21). It is therefore important to consider reducing the delay before surgery to reduce further the dropout rate.
The majority of oncological drop-outs in our series concerned CRLMs (20/28), highlighting a limitation in patient selection. Some authors consider that this interval between embolization and surgery allows to select patients who would really benefit from surgery from those with excessively aggressive disease (31). On the other hand, tumor progression can also mean that the only chance of curative resection or long-term survival is lost (32). In addition to surgical resectability criteria, it will be important to integrate CRLMs’ biological characteristics. We now have evidence that mutation status has a prognostic impact on patients with CRLM, with RAS and BRAF mutations associated with poorer overall survival and recurrence-free survival compared with wild-type patients (32-34). This is even more pronounced when the serum level of circulating tumour DNA (ctDNA) is studied. This is directly correlated with recurrence risk following liver resection (35). In the future, these data will be essential for a better understanding of disease progression and better patient selection who will really benefit from major hepatectomy with the aim of personalized medicine.
The post-hepatectomy severe complication rate was 21.1%, similar to those of previous retrospective comparative studies on hepatectomy after LVD (Clavien-Dindo ≥ IIIA, 8–35%) (17,18,23,28,36). In these studies, the rate ranged from 10–37% after PVE. Our rate is also similar to the severe complication rates observed in the most recent ALPPS studies (37,38). Indeed, Chebaro et al. (25) compared the severe complication rates after LVD and ALPPS; these were 21.9% and 22.6% respectively, thus, not significantly different. This also highlights the reductions of ALPPS morbidity and mortality apparent in the most recent studies thanks to a very good patient selection and indication, which is not yet achieved for LVDs.
PHLF was a major cause of morbidity and mortality in our series, with severe grade C PHLF being observed in 11 patients (6.8%), of whom 5 died (3.1%). PHLF was the prime cause of 90-day mortality in our study. Unsurprisingly, patients with clinically relevant PHLF (grade B/C) had a higher proportion of CCA, segment IV-extended hepatectomies, and a lower reassessment FLR%. However, the mean reassessment volumetry of 37%±10% which is usually considered sufficient to avoid a risk of PHLF. The risk of PHLF post-LVD varied considerably in previous studies: Laurent et al. (18) observed no post-LVD PHLF, Heil et al. (27) 11%, and Panaro et al. (23) 23% in small retrospective series. This raises a question in terms of liver functional assessment; volumetry alone is not enough is not sufficient to completely prevent the risk of PHLF. Volumetry aside, there are several clinical, biological, and image-guided methods by which to assess preoperative liver function and the PHLF risk, and these could be used to anticipate such risk, even after LVD. The Child-Pugh and MELD (Model of End Stage Liver Disease) scores do not appropriately measure this risk (39) for patient without cirrhosis, even if, in clinical practice, they provide an interesting indication of the possibility of liver failure. However, the combination of routine liver biology with new scores that consider the albumin, bilirubin, and platelet levels, such as the aminotransferase to platelet ratio index (APRI) and albumin-bilirubin (ALBI) grade scores, effectively screen for the PHLF risk (40-43). An algorithm for a smartphone application has been developed to facilitate the routine use of such scores (44). Liver scintigraphy assesses liver function using galactosyl human serum albumin (GSA) or 99mTc-labeled mebrofenin and can identify patients at risk of PHLF both before and after PVE or LVD, especially patients with borderline FLR volumes (45-47).
It will be interesting to read the results of the prospective randomized HYPER-LIV01 trial (48), which will compare the FLR% and liver function revealed by mebrofenin liver scintigraphy by PVE and LVD status. Given the imperfect correlation between liver volume and function, a concomitant functional assessment is essential to reduce further the risk of PHLF. The lack of functional assessment is a limitation of our study but, given the retrospective nature of the work, biological scores and nuclear images were not available.
When we looked closer at the five patients who died of PHLF grade C, it seemed that both the initial (17%) and post-LVD (26%) FLR were lower than those of other patients, and the proportions of CCA (3/5) and a need for extended right hepatectomy (4/5) higher. According to our initial post LVD volumetric criteria for validating liver resection (>30% or >40% depending on the quality of the liver parenchyma), these patients should certainly not have undergone major hepatectomy after reassessment. It is necessary to understand the causes of insufficient hypertrophy and its mechanisms. It would have been relevant to check whether portal embolization had been incomplete because of a spared sector or too proximal embolization. In these cases, it is possible to complete the procedure or to additionally embolize MHV if the right hepatectomy is to be extended. If the LVD is complete, the liver parenchyma must be analyzed to look for a possible cause of insufficient hypertrophy. The presence of liver fibrosis (49,50), chemotherapy associated liver injury (CALI) (51,52), steatosis (53), viral hepatitis (54) or even portal hypertension (55) or cholestatic jaundice (56) are limiting factors for hypertrophy. These factors must be identified (biological tests, liver biopsy, imaging), anticipated and corrected whenever possible to optimize hypertrophy. These data are missing from this registry and represent a limitation in understanding insufficient hypertrophy for these 5 patients who died of PHLF.
Different strategies have been described as a curative “last resort” for these patients whose hypertrophy is insufficient even after LVD to consider major hepatectomy. Salvage ALPPS has been described as a feasible and effective alternative to failed PVE with results similar to usual ALPPS in selected patients with CRLM and small retrospective studies (57-59). Salvage ALPPS after failed LVD was described in the series by Chebaro et al. (25) for only 6 patients but was feasible and efficient. Some expert centers are offering innovative vessel-guided hepatic parenchymal sparing techniques for CRLM (60), thanks to the recognition and intraoperative sparing of communicating veins and the concept of vascular R1 offering local oncological control similar to R0 resection (61,62). These new techniques, like transverse hepatectomies, minimize the risk of PHLF and make it easier to perform iterative resections (63,64). Finally, liver transplantation is becoming an increasingly credible alternative in the management of CRLM. This approach has been described for unresectable liver metastases in highly selected patients (65,66), and is currently being studied in the randomized TRANSMET trial.
There was no long-term evaluation of oncological outcomes in our register which constitute a limit, which would be interesting, in particular in terms of CRLM. It will be important to study in detail the forthcoming results of Pecquenard et al. (67), who are comparing the overall and recurrence-free survival of patients managed for CRLM who underwent LVD or ALPPS. In the preliminary results, despite a resectability rate in favor of ALPPS (98.9% vs. 84.3%, P<0.0001), there was no significant difference in terms of overall survival and recurrence-free survival in the context of CRLM.
Our register has several limitations, firstly because of its retrospective nature. It presents some missing data, especially for the types of preoperative treatments and their impact on liver regeneration, or on initial characteristics of each patient’s liver parenchyma quality. Unfortunately, we have too many missing data on the type and size of vascular plugs for each patient, or whether the radiologist performed selective embolization of venous collaterals between FLR and resected liver. This information would have been of interest in understanding mechanisms of hypertrophy insufficiency or LVD complications.
However, it reflects current LVD practice in France.
The heterogeneity of patients’ initial characteristics highlights the lack of standardization of LVD, the indication and use of which vary greatly between centers. Harmonization of practices would reduce the risk of complications and loss of opportunity for patients.
Moreover, there was no functional assessment in this registry, although this measure will be essential in order to reduce PHLF rates. However, several patients from this registry were included in the French HYPERLIV-01 randomised trial, in which 99mmebrofenin scintigraphy was performed.
Conclusions
LVD is becoming increasingly common in liver surgery centers. LVD is safe and feasible with 100% success rate in this study and 2.6% of severe complications. LVD is effective, inducing rapid and significant hypertrophy, thus increasing the liver resectability rate, especially of patients at risk of PVE failure. However, the indications for LVD and the roles that LVD plays need to be standardized more comprehensively by harmonizing practices among centers. The drop-out rate, more often by oncological progression, is lower than that observed in PVE series, but remains significant. The rate of clinically significant PHLF remains significant despite satisfactory volumetric reassessment; concomitant liver reserve functional evaluation will be essential for future studies (HYPERLIV01). The effects of LVD on long-term oncological outcomes, especially CRLM, must be compared in randomized trials to those of other liver hypertrophy techniques (ALPPS and PVE).
Acknowledgments
The English in this document has been checked by at least two professional editors, both native speakers of English. For a certificate, please see: http://www.textcheck.com/certificate/2nplY3.
Funding: None.
Footnote
Reporting Checklist: The authors have completed the STROCSS reporting checklist. Available at https://hbsn.amegroups.com/article/view/10.21037/hbsn-24-315/rc
Data Sharing Statement: Available at https://hbsn.amegroups.com/article/view/10.21037/hbsn-24-315/dss
Peer Review File: Available at https://hbsn.amegroups.com/article/view/10.21037/hbsn-24-315/prf
Conflicts of Interest: All authors have completed the ICMJE uniform disclosure form (available at https://hbsn.amegroups.com/article/view/10.21037/hbsn-24-315/coif). The authors have no conflicts of interest to declare.
Ethical Statement: The authors are accountable for all aspects of the work in ensuring that questions related to the accuracy or integrity of any part of the work are appropriately investigated and resolved. The study was conducted in accordance with the Declaration of Helsinki (as revised in 2013). Inform consent was obtained at the surgical consultation or during hospital stay. This research was retrospectively registered and validated by Bordeaux University Hospital ethical committee (No. CER-BDX 2024-202).
Open Access Statement: This is an Open Access article distributed in accordance with the Creative Commons Attribution-NonCommercial-NoDerivs 4.0 International License (CC BY-NC-ND 4.0), which permits the non-commercial replication and distribution of the article with the strict proviso that no changes or edits are made and the original work is properly cited (including links to both the formal publication through the relevant DOI and the license). See: https://creativecommons.org/licenses/by-nc-nd/4.0/.
References
- Vibert E, Pittau G, Gelli M, et al. Actual incidence and long-term consequences of posthepatectomy liver failure after hepatectomy for colorectal liver metastases. Surgery 2014;155:94-105. [Crossref] [PubMed]
- Rahbari NN, Garden OJ, Padbury R, et al. Posthepatectomy liver failure: a definition and grading by the International Study Group of Liver Surgery (ISGLS). Surgery 2011;149:713-24. [Crossref] [PubMed]
- Eshkenazy R, Dreznik Y, Lahat E, et al. Small for size liver remnant following resection: prevention and management. Hepatobiliary Surg Nutr 2014;3:303-12. [Crossref] [PubMed]
- Truant S, Oberlin O, Sergent G, et al. Remnant Liver Volume to Body Weight Ratio ≥ 0.5%: A New Cut-Off to Estimate Postoperative Risks after Extended Resection in Noncirrhotic Liver. J Am Coll Surg 2007;204:22-33. [Crossref] [PubMed]
- Primavesi F, Maglione M, Cipriani F, et al. E-AHPBA-ESSO-ESSR Innsbruck consensus guidelines for preoperative liver function assessment before hepatectomy. Br J Surg 2023;110:1331-47. [Crossref] [PubMed]
- Moris D, Ronnekleiv-Kelly S, Rahnemai-Azar AA, et al. Parenchymal-Sparing Versus Anatomic Liver Resection for Colorectal Liver Metastases: a Systematic Review. J Gastrointest Surg 2017;21:1076-85. [Crossref] [PubMed]
- Adam R, Laurent A, Azoulay D, et al. Two-stage hepatectomy: A planned strategy to treat irresectable liver tumors. Ann Surg 2000;232:777-85. [Crossref] [PubMed]
- Makuuchi M, Thai BL, Takayasu K, et al. Preoperative portal embolization to increase safety of major hepatectomy for hilar bile duct carcinoma: a preliminary report. Surgery 1990;107:521-7.
- Abdalla EK, Barnett CC, Doherty D, et al. Extended hepatectomy in patients with hepatobiliary malignancies with and without preoperative portal vein embolization. Arch Surg 2002;137:675-80; discussion 680-1. [Crossref] [PubMed]
- Alvarez FA, Castaing D, Figueroa R, et al. Natural history of portal vein embolization before liver resection: a 23-year analysis of intention-to-treat results. Surgery 2018;163:1257-63. [Crossref] [PubMed]
- van Lienden KP, van den Esschert JW, de Graaf W, et al. Portal vein embolization before liver resection: a systematic review. Cardiovasc Intervent Radiol 2013;36:25-34. [Crossref] [PubMed]
- Wicherts DA, Miller R, de Haas RJ, et al. Long-term results of two-stage hepatectomy for irresectable colorectal cancer liver metastases. Ann Surg 2008;248:994-1005. [Crossref] [PubMed]
- Schnitzbauer AA, Lang SA, Goessmann H, et al. Right portal vein ligation combined with in situ splitting induces rapid left lateral liver lobe hypertrophy enabling 2-staged extended right hepatic resection in small-for-size settings. Ann Surg 2012;255:405-14. [Crossref] [PubMed]
- Schadde E, Raptis DA, Schnitzbauer AA, et al. Prediction of Mortality After ALPPS Stage-1: An Analysis of 320 Patients From the International ALPPS Registry. Ann Surg 2015;262:780-5; discussion 785-6. [Crossref] [PubMed]
- Schadde E, Ardiles V, Robles-Campos R, et al. Early survival and safety of ALPPS: first report of the International ALPPS Registry. Ann Surg 2014;260:829-36; discussion 836-8. [Crossref] [PubMed]
- Hwang S, Lee SG, Ko GY, et al. Sequential preoperative ipsilateral hepatic vein embolization after portal vein embolization to induce further liver regeneration in patients with hepatobiliary malignancy. Ann Surg 2009;249:608-16. [Crossref] [PubMed]
- Guiu B, Quenet F, Panaro F, et al. Liver venous deprivation versus portal vein embolization before major hepatectomy: future liver remnant volumetric and functional changes. Hepatobiliary Surg Nutr 2020;9:564-76. [Crossref] [PubMed]
- Laurent C, Fernandez B, Marichez A, et al. Radiological Simultaneous Portohepatic Vein Embolization (RASPE) Before Major Hepatectomy: A Better Way to Optimize Liver Hypertrophy Compared to Portal Vein Embolization. Ann Surg 2020;272:199-205. [Crossref] [PubMed]
- Le Roy B, Perrey A, Fontarensky M, et al. Combined Preoperative Portal and Hepatic Vein Embolization (Biembolization) to Improve Liver Regeneration Before Major Liver Resection: A Preliminary Report. World J Surg 2017;41:1848-56. [Crossref] [PubMed]
- Gruttadauria S, Di Francesco F, Miraglia R. Liver venous deprivation: an interesting approach for regenerative liver surgery. Updates Surg 2022;74:385-6. [Crossref] [PubMed]
- Guiu B, Chevallier P, Denys A, et al. Simultaneous trans-hepatic portal and hepatic vein embolization before major hepatectomy: the liver venous deprivation technique. Eur Radiol 2016;26:4259-67. [Crossref] [PubMed]
- Le Roy B, Gallon A, Cauchy F, et al. Combined biembolization induces higher hypertrophy than portal vein embolization before major liver resection. HPB (Oxford) 2020;22:298-305. [Crossref] [PubMed]
- Panaro F, Giannone F, Riviere B, et al. Perioperative impact of liver venous deprivation compared with portal venous embolization in patients undergoing right hepatectomy: preliminary results from the pioneer center. Hepatobiliary Surg Nutr 2019;8:329-37. [Crossref] [PubMed]
- Romero Ubillus WJ, Munoz J, Vekaria M, et al. Post-embolization syndrome: Outcomes regarding the type of embolization. Journal of Clinical Oncology 2011;29:abstr e14582.
- Chebaro A, Buc E, Durin T, et al. Liver Venous Deprivation or Associating Liver Partition and Portal Vein Ligation for Staged Hepatectomy?: A Retrospective Multicentric Study. Ann Surg 2021;274:874-80. [Crossref] [PubMed]
- Azoulay D, Castaing D, Krissat J, et al. Percutaneous portal vein embolization increases the feasibility and safety of major liver resection for hepatocellular carcinoma in injured liver. Ann Surg 2000;232:665-72. [Crossref] [PubMed]
- Heil J, Korenblik R, Heid F, et al. Preoperative portal vein or portal and hepatic vein embolization: DRAGON collaborative group analysis. Br J Surg 2021;108:834-42. [Crossref] [PubMed]
- Kobayashi K, Yamaguchi T, Denys A, et al. Liver venous deprivation compared to portal vein embolization to induce hypertrophy of the future liver remnant before major hepatectomy: A single center experience. Surgery 2020;167:917-23. [Crossref] [PubMed]
- Shindoh J, Truty MJ, Aloia TA, et al. Kinetic growth rate after portal vein embolization predicts posthepatectomy outcomes: toward zero liver-related mortality in patients with colorectal liver metastases and small future liver remnant. J Am Coll Surg 2013;216:201-9. [Crossref] [PubMed]
- Hoekstra LT, van Lienden KP, Doets A, et al. Tumor progression after preoperative portal vein embolization. Ann Surg 2012;256:812-7; discussion 817-8. [Crossref] [PubMed]
- Giraudo G, Greget M, Oussoultzoglou E, et al. Preoperative contralateral portal vein embolization before major hepatic resection is a safe and efficient procedure: a large single institution experience. Surgery 2008;143:476-82. [Crossref] [PubMed]
- Tanabe KK. Commentary on "Can we improve the morbidity and mortality associated with the associating liver partition with portal vein ligation for staged hepatectomy (ALPPS) procedure in the management of colorectal liver metastases?". Surgery 2015;157:204-6. [Crossref] [PubMed]
- Jones JC, Renfro LA, Al-Shamsi HO, et al. (Non-V600) BRAF Mutations Define a Clinically Distinct Molecular Subtype of Metastatic Colorectal Cancer. J Clin Oncol 2017;35:2624-30. [Crossref] [PubMed]
- Vauthey JN, Zimmitti G, Kopetz SE, et al. RAS mutation status predicts survival and patterns of recurrence in patients undergoing hepatectomy for colorectal liver metastases. Ann Surg 2013;258:619-26; discussion 626-7. [Crossref] [PubMed]
- Bolhuis K, van 't Erve I, Mijnals C, et al. Postoperative circulating tumour DNA is associated with pathologic response and recurrence-free survival after resection of colorectal cancer liver metastases. EBioMedicine 2021;70:103498. [Crossref] [PubMed]
- Heil J, Schadde E. Simultaneous portal and hepatic vein embolization before major liver resection. Langenbecks Arch Surg 2021;406:1295-305. [Crossref] [PubMed]
- Schadde E, Schnitzbauer AA, Tschuor C, et al. Systematic review and meta-analysis of feasibility, safety, and efficacy of a novel procedure: associating liver partition and portal vein ligation for staged hepatectomy. Ann Surg Oncol 2015;22:3109-20. [Crossref] [PubMed]
- Linecker M, Björnsson B, Stavrou GA, et al. Risk Adjustment in ALPPS Is Associated With a Dramatic Decrease in Early Mortality and Morbidity. Ann Surg 2017;266:779-86. [Crossref] [PubMed]
- Wang YY, Zhong JH, Su ZY, et al. Albumin-bilirubin versus Child-Pugh score as a predictor of outcome after liver resection for hepatocellular carcinoma. Br J Surg 2016;103:725-34. [Crossref] [PubMed]
- Amptoulach S, Gross G, Sturesson C, et al. Preoperative Aspartate Aminotransferase-to-Platelet Ratio Index Predicts Perioperative Liver-Related Complications Following Liver Resection for Colorectal Cancer Metastases. Scand J Surg 2017;106:311-7. [Crossref] [PubMed]
- Andreatos N, Amini N, Gani F, et al. Albumin-Bilirubin Score: Predicting Short-Term Outcomes Including Bile Leak and Post-hepatectomy Liver Failure Following Hepatic Resection. J Gastrointest Surg 2017;21:238-48. [Crossref] [PubMed]
- Starlinger P, Ubl DS, Hackl H, et al. Combined APRI/ALBI score to predict mortality after hepatic resection. BJS Open 2021;5:zraa043. [Crossref] [PubMed]
- Pereyra D, Rumpf B, Ammann M, et al. The Combination of APRI and ALBI Facilitates Preoperative Risk Stratification for Patients Undergoing Liver Surgery After Neoadjuvant Chemotherapy. Ann Surg Oncol 2019;26:791-9. [Crossref] [PubMed]
- Santol J, Kim S, Gregory LA, et al. An APRI+ALBI Based Multivariable Model as Preoperative Predictor for Posthepatectomy Liver Failure. Ann Surg 2023; Epub ahead of print. [Crossref]
- Olthof PB, Coelen RJS, Bennink RJ, et al. (99m)Tc-mebrofenin hepatobiliary scintigraphy predicts liver failure following major liver resection for perihilar cholangiocarcinoma. HPB (Oxford) 2017;19:850-8. [Crossref] [PubMed]
- Gupta M, Choudhury PS, Singh S, et al. Liver Functional Volumetry by Tc-99m Mebrofenin Hepatobiliary Scintigraphy before Major Liver Resection: A Game Changer. Indian J Nucl Med 2018;33:277-83. [Crossref] [PubMed]
- Wang Q, Brismar TB, Gilg S, et al. Multimodal perioperative assessment of liver function and volume in patients undergoing hepatectomy for colorectal liver metastasis: a comparison of the indocyanine green retention test, (99m)Tc mebrofenin hepatobiliary scintigraphy and gadoxetic acid enhanced MRI. Br J Radiol 2022;95:20220370. [Crossref] [PubMed]
- Deshayes E, Piron L, Bouvier A, et al. Study protocol of the HYPER-LIV01 trial: a multicenter phase II, prospective and randomized study comparing simultaneous portal and hepatic vein embolization to portal vein embolization for hypertrophy of the future liver remnant before major hepatectomy for colo-rectal liver metastases. BMC Cancer 2020;20:574. [Crossref] [PubMed]
- Teh SH, Christein J, Donohue J, et al. Hepatic resection of hepatocellular carcinoma in patients with cirrhosis: Model of End-Stage Liver Disease (MELD) score predicts perioperative mortality. J Gastrointest Surg 2005;9:1207-15; discussion 1215. [Crossref] [PubMed]
- Cucchetti A, Ercolani G, Cescon M, et al. Recovery from liver failure after hepatectomy for hepatocellular carcinoma in cirrhosis: meaning of the model for end-stage liver disease. J Am Coll Surg 2006;203:670-6. [Crossref] [PubMed]
- Narita M, Oussoultzoglou E, Fuchshuber P, et al. What is a safe future liver remnant size in patients undergoing major hepatectomy for colorectal liver metastases and treated by intensive preoperative chemotherapy? Ann Surg Oncol 2012;19:2526-38. [Crossref] [PubMed]
- Nordlinger B, Sorbye H, Glimelius B, et al. Perioperative chemotherapy with FOLFOX4 and surgery versus surgery alone for resectable liver metastases from colorectal cancer (EORTC Intergroup trial 40983): a randomised controlled trial. Lancet 2008;371:1007-16. [Crossref] [PubMed]
- Reddy SK, Marsh JW, Varley PR, et al. Underlying steatohepatitis, but not simple hepatic steatosis, increases morbidity after liver resection: a case-control study. Hepatology 2012;56:2221-30. [Crossref] [PubMed]
- Marshall A, Rushbrook S, Davies SE, et al. Relation between hepatocyte G1 arrest, impaired hepatic regeneration, and fibrosis in chronic hepatitis C virus infection. Gastroenterology 2005;128:33-42. [Crossref] [PubMed]
- Chen X, Zhai J, Cai X, et al. Severity of portal hypertension and prediction of postoperative liver failure after liver resection in patients with Child-Pugh grade A cirrhosis. Br J Surg 2012;99:1701-10. [Crossref] [PubMed]
- Cherqui D, Benoist S, Malassagne B, et al. Major liver resection for carcinoma in jaundiced patients without preoperative biliary drainage. Arch Surg 2000;135:302-8. [Crossref] [PubMed]
- Enne M, Schadde E, Björnsson B, et al. ALPPS as a salvage procedure after insufficient future liver remnant hypertrophy following portal vein occlusion. HPB (Oxford) 2017;19:1126-9. [Crossref] [PubMed]
- Sparrelid E, Gilg S, Brismar TB, et al. Rescue ALPPS is efficient and safe after failed portal vein occlusion in patients with colorectal liver metastases. Langenbecks Arch Surg 2017;402:69-75. [Crossref] [PubMed]
- Ulmer TF, de Jong C, Andert A, et al. ALPPS Procedure in Insufficient Hypertrophy After Portal Vein Embolization (PVE). World J Surg 2017;41:250-7. [Crossref] [PubMed]
- Torzilli G, Garancini M, Donadon M, et al. Intraoperative ultrasonographic detection of communicating veins between adjacent hepatic veins during hepatectomy for tumours at the hepatocaval confluence. Br J Surg 2010;97:1867-73. [Crossref] [PubMed]
- Viganò L, Ravarino N, Ferrero A, et al. Prospective evaluation of accuracy of liver biopsy findings in the identification of chemotherapy-associated liver injuries. Arch Surg 2012;147:1085-91. [Crossref] [PubMed]
- Viganò L, Procopio F, Cimino MM, et al. Is Tumor Detachment from Vascular Structures Equivalent to R0 Resection in Surgery for Colorectal Liver Metastases? An Observational Cohort. Ann Surg Oncol 2016;23:1352-60. [Crossref] [PubMed]
- Procopio F, Famularo S, Branciforte B, et al. Transversal hepatectomies: Classification and intention-to-treat validation of new parenchyma-sparing procedures for deep-located hepatic tumors. Surgery 2023;173:412-9. [Crossref] [PubMed]
- Torzilli G, Procopio F, Viganò L, et al. The Liver Tunnel: Intention-to-treat Validation of a New Type of Hepatectomy. Ann Surg 2019;269:331-6. [Crossref] [PubMed]
- Hagness M, Foss A, Line PD, et al. Liver transplantation for nonresectable liver metastases from colorectal cancer. Ann Surg 2013;257:800-6. [Crossref] [PubMed]
- Varley R, Tarazi M, Davé M, et al. Liver Transplantation for Non-Resectable Liver Metastases from Colorectal Cancer: A Systematic Review and Meta-Analysis. World J Surg 2021;45:3404-13. [Crossref] [PubMed]
- Pecquenard F, Boubaddi M, Buc E, et al. Liver Venous Deprivation or ALPPS in the Treatment of Colorectal Liver Metastasis: A Comparison of Oncological Outcome. HPB 2023;25:S257-8.