A new link between innate immunity and hepatic metabolism: attractive unknown place to visit
The potential role of gut microbial products is one of the most highlighted issues in nonalcoholic steatohepatitis (NASH) and type 2 diabetes. Gut dysbiosis and increased intestinal permeability are considered to enhance the translocation of microbial products such as endotoxin (lipopolysaccharide: LPS). This in turn activates toll-like receptors (TLRs) inducing inflammatory changes in target tissues and organs. TLR signaling pathways thus build a bridge between the gut microbiome and host organs. Each TLR detects a specific pathogen-associated molecular pattern (PAMP). TLR4 detects endotoxin from Gram-negative bacteria, while TLR2 detects bacterial lipoprotein and peptidoglycan and TLR5 detects bacterial flagellin. Intracellular TLR3 and TLR9 are activated by microbe-derived nucleic acids (1). In addition to endotoxin, endogenous ligands such as free fatty acids are known to stimulate TLR4, which may predispose to systemic low-grade inflammation and insulin resistance in patients with NASH and type 2 diabetes. Hepatocyte-specific Tlr4 deletion was reported to ameliorate hepatic steatosis, inflammation and insulin resistance in mice fed a high-fat diet (HFD) (2).
Myeloid differentiation primary-response gene 88 (MyD88) works as a central adaptor molecule of TLRs except for TLR3 (3). After the binding of corresponding ligands, TLRs activate MyD88-dependent and -independent signaling pathways, which have been finely delineated by the previous studies on macrophages (4). There have been several experiments looking for benefits of TLRs or MyD88-targetted therapeutics. A brief summary of these signaling pathways (as shown in Figure 1) may help us to understand the meaning of these gene deletion studies. MyD88 recruits IL-1R-associated kinase (IRAK) 4 and IRAK1, inducing assembly of a multiple protein complex including TNFR-associated factor (TRAF) 6, TGF-β-activated kinase 1 (TAK1) and NF-kB essential modulator (NEMO). TRAF6 and MyD88-independent TRAF3, which are ubiquitinated and degraded, activate a complex of TAK1, TAK1-binding protein 1 (TAB1), TAB2, and TAB3. This phosphorylates IkB kinase (IKK) and MAP kinase including c-Jun N-terminal kinases (JNK) and p38. IKK then degrades IκBα leading to translocation of NF-κB into the nucleus. This NF-κB as well as AP-1 induced by JNK and p38 finally enhances transcription of pro-inflammatory cytokines such as TNF-α, IL-6 and IL-1β (3).
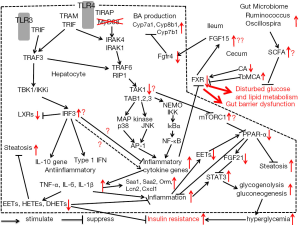
MyD88-deleted macrophages did not produce any inflammatory cytokines in response to LPS (4). Based on results in immune cells, MyD88 has been considered as a key molecule in the development of metabolic syndrome and NASH. Furthermore, MyD88 knockout mice and IRAK4 knockout mice showed profound impairment in the response to microbial components and resulting pro-inflammatory reactions (4). MyD88 deletion in the central nervous system and intestinal epithelial cells protected weight gain and glucose intolerance in mice fed HFD (5,6). It is thus worth investigating how the hepatocyte-specific MyD88 deletion affects glucose and lipid metabolism in the liver.
This year, Duparc et al. (7) answered to these questions in their manuscript in Gut, evaluating the changes in glucose, lipid and bile acids metabolism together with gut microbiota and metabolome using hepatocyte-specific MyD88-deleted mice. As a result, they found that the MyD88 deletion unexpectedly predisposed to hepatic inflammation and insulin resistance even in mice not fed on HFD. They considered that these inflammatory responses were related to the changes in gene expressions and transcriptional factor activities such as peroxisome proliferator activator receptor-alpha (PPARα), farnesoid X receptor (FXR), liver X receptors (LXRs) and STAT3, resembling those observed during diet-induced obesity. Although this study may indicate the existence of unknown linking of hepatic innate immunity and metabolism, the authors did not clearly explain why the deletion of MyD88, the supposed key player of inflammation and metabolic syndrome, conversely enhanced general inflammatory and metabolic changes.
To explain these results, we should speculate that the hepatocyte MyD88-dependent pathway may mediate anti-inflammatory responses or hepatocyte MyD88-independent pathway may have pro-inflammatory responses. Strong induction of genes involved in inflammation and acute phase response such as Saa1, Saa2, Saa3, Orm1, Orm2 and Cxcl1 in the liver may be attributed to overproductions of NF-κB-induced pro-inflammatory cytokines, although they were not determined. The comprehensive analyses of MyD88-dependent and independent downstream adapter molecules in hepatocytes may solve this question. A tentative mechanism to explain these results is added to Figure 1.
It is quite possible that MyD88-independent pathway compensates MyD88-dependent pathway in the hepatocytes with these mice. In fact, removal of MyD88 was reported to enhance TRAM-dependent pathway with enhanced activation of TRAM and IRF3 (8). In relation to the TRAM-TRIF pathway, TRIF can activate NF-κB via TRAF6 and receptor interacting protein (RIP)1 (9). This pathway activates NF-κB and induces the production of inflammatory cytokines in a delayed fashion (10). In the adipose tissue of obese mice and humans, IRF3 expression is known to be upregulated (11) and specific IRF3 knockdown prevented insulin resistance (11). IRF3 is more important compared with NF-κB, as a major regulator of adipose inflammation, maintaining systemic glucose and energy homeostasis (11). A strong inhibition of LXRs in hepatocyte-specific MyD88 deleted mice can be attributable to IRF3, because IRF3 is known to inhibit the transcriptional activity of LXR on its target promoters (12). This IRF3-mediated pro-inflammatory actions can explain the phenotype of this MyD88-deleted mice, although it is unknown if hepatic IRF3 is really upregulated in them.
Hepatocyte-specific MyD88-deleted mice (7) are characterized by depressed hepatic PPAR-α, which may aggravate hepatic steatosis, inflammation and altered insulin sensitivity. There was certainly a decrease in the gene related to the production of epoxyeicosatrienoic acids (EETs), a PPAR-α agonist and insulin sensitizer in these mice. However, is this really the only factor to suppress PPAR-α activity?
Interestingly enough, hepatocyte-specific deletion of Tak1, one of the downstream adapter molecules of MyD88 signaling, has been also proved to reveal hepatosteatosis in mice (13). In this study, hepatocyte-specific Tak1 deletion increased mTOR complex 1 (mTORC1), which is known to inhibit PPAR-α. If upstream MyD88 deletion can predispose to the inhibition of Tak1 signaling, the PPAR-α suppression with liver steatosis and inflammation may be adequately explained. Anyway, suppressed PPAR-α activity causes the decrease in FGF21 expression, inducing the elevation of serum triglyseride and glucose levels (14). FGF21 suppression may enhance STAT3 and disturb hepatic glucose metabolism in these mice, because hepatic FGF21 knockdown increases gluconeogenesis and glycogenolysis by activation of glucose-6-phosphatase and phosphoenolpyruvate carboxykinase via the STAT3/SOCS3 pathway (15). If MyD88-dependent pathway really transduces anti-inflammatory signal by preserving TAK1 and PPAR-α in the steady-state hepatocyte, MyD88 deletion may shift the hepatocyte to the pro-inflammatory state as Duparc et al. observed (7).
The effect of MyD88 on bile acids, gut microbiota and metabolome reported by Duparc et al. (7) has certainly indicated a new link between the innate immunity and metabolism in hepatocytes, although there still remain lots of unexplained phenomena. As for bile acids metabolism, the markedly increased Fgf15 mRNA expression in the ileum was quite contradictory to depressed Fgfr4 mRNA and increased Cyp7a1 mRNA expressions, because Fgf15 is known to stimulate Fgfr4 leading to suppressions of Cyp7a1 and bile acid synthesis. Duparc et al. (7) described this as an altered negative feedback loop of bile acid synthesis and I myself cannot find any adequate explanation for this phenomenon. Increased Cyp7a1 mRNA can be explained by the decrease in cholic acid, which may in turn reduce FXR activity resulting in disturbed glucose and lipid metabolism.
The fact that the genetic deletion of MyD88 in hepatocytes changes the gut microbiota composition and their metabolomes, resembling those observed during diet-induced obesity support that the fecal microbiota may have robust adaptability to the intestinal microenvironment related to the suspected metabolic changes in hepatocyte MyD88 deletion. Increased abundance of fecal Ruminococcus and Oscillospira is considered to antagonize prediabetic and pro-inflammatory states because these taxa produce large quantities of short chain fatty acids (SCFA) which are known to decrease serum levels of glucose, insulin resistance and inflammation in the host. Although the mechanism how the gut microbiome can sense the deranged MyD88 signaling is undetermined in the study, this may suggest that the gut microbiome can compensate for the nutritional state.
This study shows that the MyD88 pathway of hepatocytes may transduce undefined anti-inflammatory signaling, which has not been found in macrophages or intestinal epithelial cells. It is quite reasonable to consider that this anti-inflammatory force is counterbalanced by pro-inflammatory force in hepatocytes in an unstimulated steady state. This balance may be shifted to pro-inflammatory as a whole, when hepatocyte TLR4 is strongly stimulated by LPS, which may explain the contradictory results between hepatocyte Tlr4 deletion and MyD88 deletion.
Taken together
Acknowledgements
None.
Footnote
Conflicts of Interest: The author has no conflicts of interest to declare.
References
- Tsujimoto H, Ono S, Mochizuki H. Role of translocation of pathogen-associated molecular patterns in sepsis. Dig Surg 2009;26:100-9. [Crossref] [PubMed]
- Jia L, Vianna CR, Fukuda M, et al. Hepatocyte Toll-like receptor 4 regulates obesity-induced inflammation and insulin resistance. Nat Commun 2014;5:3878. [Crossref] [PubMed]
- Seki E, Schnabl B. Role of innate immunity and the microbiota in liver fibrosis: crosstalk between the liver and gut. J Physiol 2012;590:447-58. [Crossref] [PubMed]
- Takeda K, Akira S. TLR signaling pathways. Semin Immunol 2004;16:3-9. [Crossref] [PubMed]
- Kleinridders A, Schenten D, Könner AC, et al. MyD88 signaling in the CNS is required for development of fatty acid-induced leptin resistance and diet-induced obesity. Cell Metab 2009;10:249-59. [Crossref] [PubMed]
- Everard A, Geurts L, Caesar R, et al. Intestinal epithelial MyD88 is a sensor switching host metabolism towards obesity according to nutritional status. Nat Commun 2014;5:5648. [Crossref] [PubMed]
- Duparc T, Plovier H, Marrachelli VG, et al. Hepatocyte MyD88 affects bile acids, gut microbiota and metabolome contributing to regulate glucose and lipid metabolism. Gut 2017;66:620-32. [Crossref] [PubMed]
- Selvarajoo K, Takada Y, Gohda J, et al. Signaling flux redistribution at toll-like receptor pathway junctions. PLoS One 2008;3:e3430. [Crossref] [PubMed]
- Brown J, Wang H, Hajishengallis GN, et al. TLR-signaling networks: an integration of adaptor molecules, kinases, and cross-talk. J Dent Res 2011;90:417-27. [Crossref] [PubMed]
- Bagchi A, Herrup EA, Warren HS, et al. MyD88-dependent and MyD88-independent pathways in synergy, priming, and tolerance between TLR agonists. J Immunol 2007;178:1164-71. [Crossref] [PubMed]
- Kumari M, Wang X, Lantier L, et al. IRF3 promotes adipose inflammation and insulin resistance and represses browning. J Clin Invest 2016;126:2839-54. [Crossref] [PubMed]
- Castrillo A, Joseph SB, Vaidya SA, et al. Crosstalk between LXR and toll-like receptor signaling mediates bacterial and viral antagonism of cholesterol metabolism. Mol Cell 2003;12:805-16. [Crossref] [PubMed]
- Inokuchi-Shimizu S, Park EJ, Roh YS, et al. TAK1-mediated autophagy and fatty acid oxidation prevent hepatosteatosis and tumorigenesis. J Clin Invest 2014;124:3566-78. [Crossref] [PubMed]
- Feingold KR, Grunfeld C, Heuer JG, et al. FGF21 is increased by inflammatory stimuli and protects leptin-deficient ob/ob mice from the toxicity of sepsis. Endocrinology 2012;153:2689-700. [Crossref] [PubMed]
- Wang C, Dai J, Yang M, et al. Silencing of FGF-21 expression promotes hepatic gluconeogenesis and glycogenolysis by regulation of the STAT3-SOCS3 signal. FEBS J 2014;281:2136-47. [Crossref] [PubMed]