The antiapoptotic effects of different doses of β-carotene in chronic ethanol-fed rats
Introduction
It is known that long-term excessive ethanol consumption induces alcoholic liver disease (ALD). The pathogenesis of ALD is a complex process and is not completely understood. Ethanol-induced liver injury may be associated with increased oxidative stress and apoptotic cell death in the liver which may lead to ALD (1,2). During ethanol metabolism, reactive oxygen species (ROS) generation increases through activation of the xanthine oxidase, cytochrome P450 2E1 (CYP2E1), and NADPH oxidase complex. ROS generation causes oxidative stress and free radical-mediated tissue damage, and is thought to be involved in the progression of ethanol-induced liver injury (1,3).
Ethanol consumption might induce apoptosis in the liver. It was pointed out that apoptotic cells are high in liver biopsies from ALD patients (4), and hepatic apoptosis increased in rats after ethanol treatment (5). Oxidative stress and inflammatory cytokines are thought to play important roles in the pathogenesis of hepatocellular apoptosis (6). Ethanol may induce ROS formation and cause a mitochondrial membrane permeability transition. Then, cytochrome c is released from the damaged mitochondria, followed by caspase activation, leading to cell apoptosis. In addition, ethanol-induced apoptosis is associated with death receptor-mediated apoptosis. Ethanol may upregulate the Fas/Fas-ligand and tumor necrosis factor (TNF) receptor 1/TNF-α systems and lead to apoptosis (2).
β-Carotene is a carotenoid which acts as a vitamin A precursor and has antioxidant ability by scavenging free radicals and quenching singlet oxygen (7). However, clinical studies found that high-dose β-carotene supplementation (20-30 mg/day) might have harmful effects in smokers (8,9). β-Carotene might act as an antioxidant that inhibits lipid peroxidation or as a prooxidant at high concentrations and at high partial pressures of oxygen (10). It was reported that the ethanol-induced hepatic depletion of vitamin A can be corrected by 30-60 mg β-carotene supplementation per day (11). In addition, ethanol-induced lipid peroxidation was inhibited in ethanol-drinking rats that received a natural β-carotene isomer mixture of Dunaliella bardawil (12). However, excess β-carotene supplementation (30-45 mg/1,000 kcal diet) might aggravate ethanol-induced liver damage (13). Therefore, β-carotene should be administered cautiously in an animal experimental model of ALD.
In our previous study, we found that β-carotene supplementation prevented ethanol-induced liver damage and increased the reduced glutathione (GSH) concentration in rats (14). In addition, 1 µM β-carotene exhibited antioxidant and antiapoptotic properties and prevented ethanol-induced cytotoxicity in primary hepatocytes (15). However, the use of β-carotene supplementation in ALD studies should be carefully considered, as it is still unknown whether high-dose β-carotene supplementation is harmful. Using that information as a basis, the objective of the present study was to investigate the effects of different doses of β-carotene supplementation on the antioxidant capacity and hepatic apoptosis in rats chronically fed ethanol.
Materials and methods
Animals and diets
Six-week-old male Wistar (BioLASCO Taiwan, Taipei, Taiwan) rats were used in this study. Rats were housed in an air-conditioned room at 23±2 °C with 50-70% humidity and a 12-h light-dark cycle. After 1 week of acclimation, rats were divided into six groups (n=10) according to similar plasma aspartate transaminase (AST) and alanine transaminase (ALT) activities. The six groups were as follows: C (control liquid diet), CLB [control liquid diet with β-carotene supplementation at 0.52 mg/kg body weight (BW)/day], CHB (control liquid diet with β-carotene supplementation at 2.6 mg/kg BW/day), E (ethanol liquid diet), ELB (ethanol liquid diet with β-carotene supplementation at 0.52 mg/kg BW/day), and EHB (ethanol liquid diet with β-carotene supplementation at 2.6 mg/kg BW/day). The lower dose of β-carotene supplementation (0.52 mg/kg BW/day) was selected according to our previous study (14). The liquid diets were prepared freshly daily and modified from Lieber and DeCarli (16). The ethanol liquid diet provided 18.23% of total calories as protein, 21.70% as fat, 25.07% as carbohydrates, and 35% as ethanol. The control liquid diet provided an isocaloric diet in which the ethanol was replaced with corn starch. β-Carotene was given as Solatene® (10% β-carotene in beadlets, Hoffmann-La Roche, South San Francisco, CA, USA). All procedures were approved by the Institutional Animal Care and Use Committee of Taipei Medical University.
Sample preparation
Rats were anesthetized and sacrificed after feeding them the respective diets for 12 weeks. Blood samples were collected in heparin-containing tubes and were centrifuged to separate the plasma (1,200× g for 15 min at 4 °C). All plasma samples were stored at –80 °C until being assayed. Liver tissues were rapidly excised and stored at –80 °C for further analysis. One gram of liver tissue was homogenized in 4 mL buffer (0.25 mM sucrose, 10 mM Tris-HCl, and 0.25 mM phenylmethylsulfonyl fluoride; pH 7.4). The homogenate was then centrifuged at 10,000× g for 15 min at 4 °C. The supernatant was analyzed for the reduced glutathione (GSH)/oxidized glutathione (GSSG) ratio and lipid peroxide levels.
Measurements of plasma AST and ALT activities
To investigate the liver function during the experimental period, plasma AST and ALT activities were measured with a Beckman Synchron LXTM system (Beckmen Coulter, Brea, CA, USA) at 340 nm.
Histological examination
Liver tissues were fixed in 10% formaldehyde and embedded in paraffin. Paraffin sections were processed for histological examination according to three kinds of histopathological stains: hematoxylin-eosin (H&E), Masson, and silver stains. A semiquantitative histological evaluation was carried out by a pathologist blinded to the treatment groups. The grading of H&E stain ranged from 0 to 4 where 0 is absent, 1 is a trace, 2 is mild, 3 is moderate, and 4 is severe. The scale for semiquantitation of hepatic tissue fibrosis (Masson and silver stains) was as follows: 0 means no collagen; 1 means the existence of collagen but no septal formation; 2 means the existence of collagen and a septum, but no connective tissue; 3 means the existence of collagen with a few thin connective tissue septa; and 4 means the existence of collagen with thick connective tissue septa.
Measurement of the GSH/GSSG ratio
The GSH concentration was measured spectrophotometrically at 405 nm according to the method of Tietze (17), and the GSSG concentration was measured spectrophotometrically at 405 nm according to the method of Griffith (18). Finally, the ratio of GSH/GSSG was calculated as (GSH –2GSSG)/(GSSG).
Measurement of lipid peroxidation
Lipid peroxidation was determined by measuring the concentration of thiobarbituric acid reactive substances (TBARS) in the liver with minor modifications (19). Briefly, 20 µL of liver homogenates or 1,1,3,3-tetramethoxypropane (TMP; as the standard) was shaken with 800 µL of 0.22% H2SO4 in a 2-mL centrifuge tube. Then, 100 µL of 10% phosphotungstic acid and 200 µL 0.67% TBA were added to the mixture, mixed well, and warmed for 60 min in a boiling-water bath followed by rapid cooling. This was shaken into 600 µL of a n-butyl-alcohol layer in a separation tube, and then measured fluorometrically (at respective excitation and emission wavelengths of 531 and 590 nm) using a Wallac Victor-2 1420 Multilabel Counter (Perkin-Elmer, Waltham, MA, USA).
Measurement of TNF-α
Liver tissue (0.5 g) was homogenized in 1.5 mL of ice-cold buffer [50 mM Tris (pH 7.2), 150 mM NaCl, and 1% Triton-X] plus 0.1% protease inhibitor. The homogenate was then shaken on ice for 90 min. After shaking, the homogenates were centrifuged at 3,000× g at 4 °C for 15 min. The supernatants were collected for subsequent analysis.
Plasma and hepatic TNF-α concentrations were measured using enzyme-linked immunosorbent assay (ELISA) kits. Plasma and hepatic TNF-α concentrations were determined using a rat TNF-α/TNFSF1A kit (R&D Systems, Minneapolis, MN, USA). Assays of samples and standards were simultaneously conducted according to the assay kit instructions. The optical density was read at 450 nm for all cytokines using a microplate reader (Molecular Devices, Sunnyvale, CA, USA).
Western blot analysis
To determine expressions of CYP2E1, Fas ligand, Bcl-2, Bcl-xL, cytochrome c, caspase-8, -9, and -3 in the liver, a Western blot analysis was performed. For CYP2E1 expression analysis, liver tissues were homogenized in 10 volumes of ice-cold buffer (0.25 M sucrose, 10 mM Tris-HCl, and 0.25 mM phenylmethylsulfonyl fluoride; pH 7.4). The homogenates were centrifuged at 17,000× g for 20 min at 4 °C. The supernatant was centrifuged at 105,000× g for 60 min at 4 °C to separate the microsomes. The microsomal pellet was dissolved in 50 mM potassium phosphate buffer containing 1 mM EDTA and 1 mM DTT (pH 7.4). Total liver protein isolated from tissues was homogenized in lysis buffer [50 mM Tris HCl at pH 7.5, 150 mM NaCl, 1% NP-40, 1% sodium deoxycholate, and 0.1% sodium dodecylsulfate (SDS)] containing a protease inhibitor cocktail (Sigma-Aldrich, St. Louis, MO, USA). The homogenates were centrifuged at 10,000× g for 15 min, and the supernatant was used for analysis of Fas ligand, Bcl-2, Bcl-xL, cytochrome c, caspase-8, -9, and -3.
Total liver proteins and microsomal protein were separated by 10% SDS polyacrylamide gel electrophoresis (PAGE). Then, the proteins were electroblotted onto a polyvinylidene difluoride (PVDF) transfer membrane (Amersham Biosciences, Little Chalfont, Bucks, UK). These blots were incubated with a mouse monoclonal anti-rat CYP2E1 antibody (Oxford Biomedical Research, Oxford, MI, USA), a polyclonal anti-rat Fas ligand antibody (Chemicon International, Temecula, CA, USA), a monoclonal anti-Bcl-2 antibody (Sigma-Aldrich), a monoclonal anti-Bcl-xL antibody (Sigma-Aldrich), an anti-cytochrome c antibody (Sigma-Aldrich), a caspase-8, -9, -3 antibody (Santa Cruz Biotechnology, Santa Cruz, CA, USA), and a mouse anti-actin monoclonal (Chemicon International) antibody.
Finally, the blot was treated with goat anti-mouse immunoglobulin G (IgG)-horseradish peroxidase (HRP) (Chemicon International) or goat anti-rabbit IgG-HRP (Santa Cruz Biotechnology), and specific bindings of antibodies were detected using a Western Lightning kit (PerkinElmer Lifesciences, Boston, MA, USA). The bands were quantified using Image-Pro Plus 4.5 software analysis.
Statistical analysis
All data are expressed as the mean ± standard error of the mean (SEM). A two-way analysis of variance (ANOVA) followed by Fisher’s test was used to determine statistical differences among groups using SAS software vers. 8.0 (SAS Institute, Cary NC, USA). Statistical significance was assigned at the P<0.05 level.
Results
The average energy intake in the six groups was as follows (mean ± SEM): C group (81.0±2.7 kcal/day), CLB group (81.1±2.9 kcal/day), CHB group (78.8±2.5 kcal/day), E group (83.7±3.2 kcal/day), ELB group (82.1±3.7 kcal/day), and EHB group (77.2±3.3 kcal/day). The average ethanol intake levels in the groups consuming ethanol were as follows: E group (4.2±0.2 g/day), ELB group (4.1±0.2 g/day), and EHB group (3.9±0.2 g/day). There were no significant differences in the energy or ethanol intake levels among these groups.
Results of the BW change and the relative liver weight are shown in Table 1. BW changes of the E, ELB, and EHB groups were significantly lower than that in the C group (P<0.05). The relative liver weights in the E and ELB groups were significantly higher than that in the C group (P<0.05). And, the relative liver weight in the EHB group was significantly lower than that in the E group E (P<0.05).
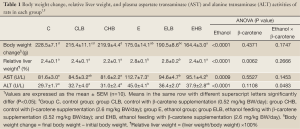
Full Table
The effects of β-carotene on plasma AST and ALT activities in ethanol-fed rats are shown in Table 1. Plasma AST and ALT activities in the E group were significantly higher than those in the C group (P<0.05) at the end of experiment. However, the plasma AST and ALT activities in the ELB group were significantly lower than those in the E group (P<0.05). The AST activity in the EHB group was also lower than that in the E group (P<0.05).
Figure 1 shows representative photomicrographs of liver samples in the six groups. Results of the semiquantitative histological assessment are given in Table 2. The score of fatty change in the E group was significantly higher than that in the C group (P<0.05). There were no significant difference in the inflammation or fibrosis scores in groups C and E. However, the inflammation and fibrosis scores of Masson staining in the EHB group were significantly lower than those in group E (P<0.05).
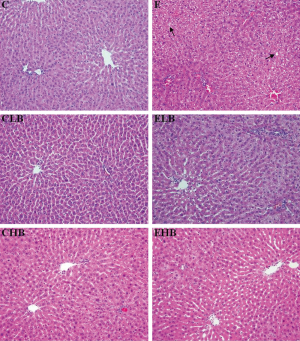
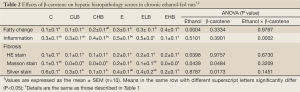
Full Table
The effects of β-carotene on TNF-α concentrations in plasma and liver tissues are shown in Table 3. Results of TNF-α concentrations in plasma and liver tissues in the various group were similar. Plasma and hepatic TNF-α levels in the E group were significantly higher than those in the C group (P<0.05); however, they were significantly lower in the EHB group compared to those in the E group (P<0.05). Furthermore, a dose-response effect of β-carotene supplementation on decreasing the TNF-α concentration was observed (P<0.05).
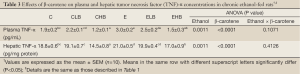
Full Table
The antioxidant status in the liver was evaluated by the TBARS concentration, GSH/GSSG ratio, and CYP2E1 expression. Results of the hepatic TBARS concentration and GSH/GSSG ratio are given in Table 4. The TBARS concentration in the E group was significantly higher than that in the C group (P<0.05); in addition, concentrations were significantly lower in the ELB and EHB groups compared to that in the E group (P<0.05). There was no significant difference in the GSH/GSSG ratio between groups C and E. GSH/GSSG ratios in the E and EHB groups were significantly lower than those in the CLB and CHB groups (P<0.05). Figure 2 shows the outcome of protein expressions in the liver. As Figure 2 shows, CYP2E1 expression significantly increased (P<0.05) in the E group. However, CYP2E1 expression in the ELB group was significantly lower than that in the E group (P<0.05).
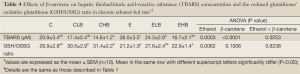
Full Table
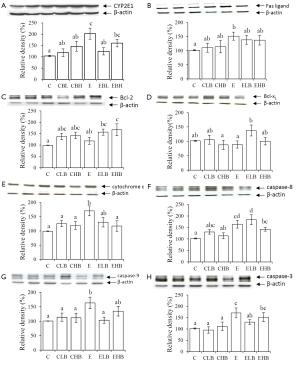
To determine the molecular mechanism effecting apoptosis in liver tissues, we analyzed protein expressions of Fas ligand, Bcl-2, Bcl-xL, cytochrome c, caspase-8, -9, and -3 by Western blotting (Figure 2). Expressions of these proteins in the CLB and CHB groups showed no significant difference compared to those in the C group. Expressions of Fas ligand, cytochrome c, caspase-8, -9, and -3 were significantly higher in the E group compared to those in the C group (P<0.05). However, caspase-9 and -3 expressions in the ELB group and cytochrome c expression in the EHB group were significantly lower than those in the E group (P<0.05). In addition, Bcl-xL expression in the ELB group and Bcl-2 expression in the EHB group were significantly lower than those of in the E group (P<0.05).
Discussion
In the present study, rats in each group were fed isocaloric diets. BW changes, relative liver weights, and plasma AST, ALT activities in rats fed the normal liquid diet supplemented with β-carotene did not differ compared to the control group (Table 1). This reveals that two doses of β-carotene supplementation did not affect the growth of rats and had no adverse effects in the present model. The BW change was significantly lower, and the relative liver weight was significant higher in the ethanol-fed group compared to the control group (Table 1). The results are consistent with our previous study (14). Plasma AST and ALT activities are markers of ethanol-induced liver injury. The results showed that plasma AST and ALT activities significantly increased in rats of E group (Table 1). In addition, hepatic fatty change was seen from the histopathological examination (Table 2) in the E group. The present results indicate that ethanol consumption led to liver injury. However, plasma AST and ALT activities in the ELB group and AST activity in the EHB group were significantly lower than the control (Table 1). According to these results, β-carotene might exhibit a hepatoprotective effect against ethanol-induced liver injury in rats.
It was reported that oxidative stress plays an important role in the progression of ethanol-induced liver injury (1). In the present study, lipid peroxidation and hepatic CYP2E1 expression increased in ethanol-fed rats (Table 4, Figure 2). Ethanol might increase levels of CYP2E1 which generates ROS and contributes to oxidative stress during ethanol metabolism (20). It was also reported that a significant correlation between CYP2E1 expression and lipid peroxidation was found in ethanol-fed rats (21). Therefore, results of the present study indicate that oxidative stress increased in rats after chronic ethanol feeding. The present results also showed that lipid peroxidation decreased in the ELB and EHB groups, and CYP2E1 expression was lower in the ELB group (Table 4, Figure 2). It was reported that β-carotene supplementation protects against ferric nitrilotriacetate-induced oxidative stress and lipid peroxidation in rats (22). It was also reported that inhibition of CYP2E1 expression can improve ethanol-induced liver injury in rats (23). We speculated that β-carotene quenches singlet oxygen (7) or decreases CYP2E1-induced oxidative stress by inhibiting CYP2E1 expression and thereby inhibits ethanol-induced lipid peroxidation in rats.
To evaluate the inflammatory response in ethanol-fed rats with β-carotene supplementation, we analyzed plasma and hepatic TNF-α levels in rats in the present study. Results show that plasma and hepatic TNF-α levels significantly increased in the E group (Table 3). Chronic ethanol exposure activates Kupffer cells by lipopolysaccharide (LPS), leading to the production of inflammatory mediators such as TNF-α and interleukin (IL)-1β that might contribute to necrosis and apoptosis of hepatocytes (24). In addition, oxidative stress induced by CYP2E1 might promote activation of nuclear factor (NF)-κB that increases TNF-α production by Kupffer cells (1). Our results showed that plasma and hepatic TNF-α levels in the EHB group were significantly lower than those in the E group. A previous study showed that 2 µM β-carotene inhibited TNF-α secretion in phorbol-12-myristate-13-acetate (PMA)-stimulated HL-60 cells (25). In addition, an intraperitoneal injection of β-carotene (10 mg/kg) inhibited TNF-α production in LPS-administrated mice through suppression of IκB degradation and subsequent NF-κB activation and inflammatory gene expression (26). In the present study, β-carotene supplementation decreased the production of TNF-α, thus decreasing ethanol-induced inflammation. Those results are consistent with the inflammation scores in the histopathological exam (Table 2). However, further studies are needed to clarify the possible mechanism of β-carotene’s anti-inflammatory activity.
To investigate the effects of β-carotene on hepatic apoptosis in ethanol-fed rats, we analyzed the expressions of proteins associated with apoptosis in the liver. Previous studies reported that hepatic apoptosis increased in mice and rats chronically fed ethanol (27). There are two signaling pathways leading to hepatic apoptosis in ALD: death receptor-mediated apoptosis and mitochondrion-mediated apoptosis (2,3). Based on our results, the hepatic TNF-α level and expressions of Fas ligand and caspase-8 increased in ethanol-fed rats (Figure 2), suggesting that ethanol consumption might induce hepatic apoptosis through the death receptor-mediated pathway. In addition, the present results also showed that expressions of hepatic CYP2E1, cytochrome c, caspase-9, and -3 increased in ethanol-fed rats. We speculated that ethanol-induced oxidative stress might cause mitochondrial dysfunction causing cytochrome c to be released from mitochondria. Then, caspase-9 and -3 are activated leading to hepatic apoptosis. The present results also showed that hepatic Bcl-2 expression increased and cytochrome c expression decreased in the EHB group. Hepatic Bcl-xL expression increased and caspase-9 and -3 expressions decreased in the ELB group (Figure 2). Bcl-2 and Bcl-xL belong to the bcl-2 family of proteins which are located in the mitochondrial outer membrane and act as inhibitors of apoptosis (28). In addition, β-carotene decreased the generation of ROS and protected hepatocytes against apoptosis through a reduction in caspase-3 activation and inhibition of mitochondrial cytochrome c release (29). According to the present results, the hepatoprotective effect of a lower dose of β-carotene supplementation in ethanol-fed rats might be associated with inhibiting the progression of mitochondrial-mediated apoptosis. The present results also suggest that the higher dose of β-carotene supplementation might inhibit TNF-α secretion and act as an antioxidant to inhibit lipid peroxidation in rats chronically fed ethanol. Although the higher dose of β-carotene supplementation inhibited lipid peroxidation, it did not seem to be related to the antiapoptotic ability in rats fed ethanol.
Taking the present results together, we found that the different doses of β-carotene supplementation prevented ethanol-induced liver injury in ethanol-fed rats. However, there may be various protective mechanisms with the different doses of β-carotene supplementation in the present study. The differential effects of β-carotene can be explained by a variety of experimental and biological factors, such as the partial pressure of oxygen (10), the dose of β-carotene supplementation used in the experiment (12,13,30), and the digestion, absorption, and bioavailability of β-carotene (31). The partial pressure of oxygen might differ in vivo because of the distribution of tissues and organs, such as 100 mmHg in lung alveoli and 5-15 mmHg in tissues (32). Therefore, β-carotene acted at low partial pressures of oxygen in tissues under physiological conditions in the present study. In addition, Kessova et al. found that β-carotene supplementation (56.5 mg/L) potentiated CYP2E1 expression in ethanol-fed rats (30), and Leo et al. reported that high-dose β-carotene supplementation (30-45 mg/1,000 kcal diet) increased the hepatotoxicity in ethanol-fed baboons (13). Compared to those studies which used high-dose β-carotene supplementation, the doses of β-carotene supplementation used in the present study were not high (0.52 and 2.6 mg/kg BW/day). In addition, the present results showed no harmful effects in ethanol-fed rats with the higher dose of β-carotene supplementation. Thus, the different hepatoprotective effects of different doses of β-carotene supplementation might be complicated in ethanol-fed rats. Additional studies are needed to clarify whether the effects of different doses of β-carotene supplementation are associated with the metabolism and bioavailability of β-carotene in ethanol-fed rats.
In conclusion, the present results suggest that ethanol treatment causes oxidative stress to increase which could promote apoptosis in the liver, thus leading to liver injury in rats. However, the lower dose of β-carotene supplementation (0.52 mg/kg BW/day), which acted as an antioxidant, decreased oxidative stress through decreasing CYP2E1 expression and inhibiting lipid peroxidation. Moreover, it prevented ethanol-induced liver damage by inhibiting hepatic apoptosis via inhibiting caspase-9 and -3 expressions and increasing Bcl-xL expression in the liver. In addition, the higher dose of β-carotene supplementation (6.2 mg/kg BW/day) possibly prevented ethanol-induced liver damage through inhibiting lipid peroxidation and TNF-α secretion in ethanol-fed rats.
Acknowledgements
This study was supported by Cathay General Hospital (94CGH-TMU-10).
Disclosure: No financial or commercial conflicts of interests have been disclosed. All authors listed have contributed to the work and have agreed to submit the manuscript to Hepatobiliary Surgery and Nutrition.
References
- Albano E. Oxidative mechanisms in the pathogenesis of alcoholic liver disease. Mol Aspects Med 2008;29:9-16. [PubMed]
- Feldstein AE, Gores GJ. Apoptosis in alcoholic and nonalcoholic steatohepatitis. Front Biosci 2005;10:3093-9. [PubMed]
- Conde de la Rosa L, Moshage H, Nieto N. Hepatocyte oxidant stress and alcoholic liver disease. Rev Esp Enferm Dig 2008;100:156-63. [PubMed]
- Zhao M, Laissue JA, Zimmermann A. TUNEL-positive hepatocytes in alcoholic liver disease. A retrospective biopsy study using DNA nick end-labelling. Virchows Arch 1997;431:337-44. [PubMed]
- Ronis MJ, Korourian S, Blackburn ML, et al. The role of ethanol metabolism in development of alcoholic steatohepatitis in the rat. Alcohol 2010;44:157-69. [PubMed]
- Nanji AA. Apoptosis and alcoholic liver disease. Semin Liver Dis 1998;18:187-90. [PubMed]
- Fukuzawa K, Inokami Y, Tokumura A, et al. Rate constants for quenching singlet oxygen and activities for inhibiting lipid peroxidation of carotenoids and alpha-tocopherol in liposomes. Lipids 1998;33:751-6. [PubMed]
- The effect of vitamin E and beta carotene on the incidence of lung cancer and other cancers in male smokers. The Alpha-Tocopherol, Beta Carotene Cancer Prevention Study Group. N Engl J Med 1994;330:1029-35. [PubMed]
- Omenn GS, Goodman GE, Thornquist MD, et al. Risk factors for lung cancer and for intervention effects in CARET, the Beta-Carotene and Retinol Efficacy Trial. J Natl Cancer Inst 1996;88:1550-9. [PubMed]
- Young AJ, Lowe GM. Antioxidant and prooxidant properties of carotenoids. Arch Biochem Biophys 2001;385:20-7. [PubMed]
- Ahmed S, Leo MA, Lieber CS. Interactions between alcohol and beta-carotene in patients with alcoholic liver disease. Am J Clin Nutr 1994;60:430-6. [PubMed]
- Werman MJ, Ben-Amotz A, Mokady S. Availability and antiperoxidative effects of beta-carotene from Dunaliella bardawil in alcohol-drinking rats. J Nutr Biochem 1999;10:449-54. [PubMed]
- Leo MA, Kim C, Lowe N, et al. Interaction of ethanol with beta-carotene: delayed blood clearance and enhanced hepatotoxicity. Hepatology 1992;15:883-91. [PubMed]
- Lin WT, Huang CC, Lin TJ, et al. Effects of beta-carotene on antioxidant status in rats with chronic alcohol consumption. Cell Biochem Funct 2009;27:344-50. [PubMed]
- Peng HC, Chen JR, Chen YL, et al. beta-Carotene exhibits antioxidant and anti-apoptotic properties to prevent ethanol-induced cytotoxicity in isolated rat hepatocytes. Phytother Res 2010;24 Suppl 2:S183-9. [PubMed]
- Lieber CS, DeCarli LM. Animal models of chronic ethanol toxicity. Methods Enzymol 1994;233:585-94. [PubMed]
- Tietze F. Enzymic method for quantitative determination of nanogram amounts of total and oxidized glutathione: applications to mammalian blood and other tissues. Anal Biochem 1969;27:502-22. [PubMed]
- Griffith OW. Determination of glutathione and glutathione disulfide using glutathione reductase and 2-vinylpyridine. Anal Biochem 1980;106:207-12. [PubMed]
- Ohkawa H, Ohishi N, Yagi K. Assay for lipid peroxides in animal tissues by thiobarbituric acid reaction. Anal Biochem 1979;95:351-8. [PubMed]
- Dey A, Cederbaum AI. Alcohol and oxidative liver injury. Hepatology 2006;43:S63-74. [PubMed]
- Nanji AA, Zhao S, Sadrzadeh SM, et al. Markedly enhanced cytochrome P450 2E1 induction and lipid peroxidation is associated with severe liver injury in fish oil-ethanol-fed rats. Alcohol Clin Exp Res 1994;18:1280-5. [PubMed]
- Matos HR, Marques SA, Gomes OF, et al. Lycopene and beta-carotene protect in vivo iron-induced oxidative stress damage in rat prostate. Braz J Med Biol Res 2006;39:203-10. [PubMed]
- Gouillon Z, Lucas D, Li J, et al. Inhibition of ethanol-induced liver disease in the intragastric feeding rat model by chlormethiazole. Proc Soc Exp Biol Med 2000;224:302-8. [PubMed]
- Schaffert CS, Duryee MJ, Hunter CD, et al. Alcohol metabolites and lipopolysaccharide: roles in the development and/or progression of alcoholic liver disease. World J Gastroenterol 2009;15:1209-18. [PubMed]
- Yeh SL, Wang HM, Chen PY, et al. Interactions of beta-carotene and flavonoids on the secretion of pro-inflammatory mediators in an in vitro system. Chem Biol Interact 2009;179:386-93. [PubMed]
- Bai SK, Lee SJ, Na HJ, et al. beta-Carotene inhibits inflammatory gene expression in lipopolysaccharide-stimulated macrophages by suppressing redox-based NF-kappaB activation. Exp Mol Med 2005;37:323-34. [PubMed]
- Colantoni A, Idilman R, De Maria N, et al. Hepatic apoptosis and proliferation in male and female rats fed alcohol: role of cytokines. Alcohol Clin Exp Res 2003;27:1184-9. [PubMed]
- Antonsson B. Mitochondria and the Bcl-2 family proteins in apoptosis signaling pathways. Mol Cell Biochem 2004;256-257:141-55. [PubMed]
- Gumpricht E, Dahl R, Devereaux MW, et al. Beta-carotene prevents bile acid-induced cytotoxicity in the rat hepatocyte: Evidence for an antioxidant and anti-apoptotic role of beta-carotene in vitro. Pediatr Res 2004;55:814-21. [PubMed]
- Kessova IG, Leo MA, Lieber CS. Effect of beta-carotene on hepatic cytochrome P-450 in ethanol-fed rats. Alcohol Clin Exp Res 2001;25:1368-72. [PubMed]
- Maiani G, Castón MJ, Catasta G, et al. Carotenoids: actual knowledge on food sources, intakes, stability and bioavailability and their protective role in humans. Mol Nutr Food Res 2009;53 Suppl 2:S194-218. [PubMed]
- Palozza P, Serini S, Di Nicuolo F, et al. Mitogenic and apoptotic signaling by carotenoids: involvement of a redox mechanism. IUBMB Life 2001;52:77-81. [PubMed]