Circadian enhancer profiling in diet-induced obese mice reveals a critical time window for lipid-lowering therapies
The 24-hour rhythms in physiology and behavior are controlled by endogenous cellular clocks found in all cells and tissue of the body. At the molecular level, these clocks are based on interlocked transcriptional-translational feedback loops comprised of a set of clock genes and proteins (1). While the nature of the core clockwork is conserved across all tissues, the output from these clocks is highly cell type-specific and believed to be specified by tissue-specific cistromes including enhancer sites, where transcription factor binding leads to regulation of gene transcription over large genomic distances. Under normal metabolic conditions, 5–10% of active transcripts oscillate in a circadian fashion within a tissue. In the mouse, up to 50% of genes are clock-controlled in at least on tissue of the body (2).
Circadian rhythm disruption—as seen, e.g., in shift workers—is associated with an increased risk for metabolic diseases such as obesity or type-2 diabetes (3), and experiments in mice suggest that diet composition itself may affect clock function in peripheral and central tissues, disrupting daily intake rhythms and promoting overeating and weight gain (4). However, the mechanisms by which the nutrient environment impacts circadian rhythms to alter metabolic homeostasis are still poorly understood.
In a recent paper published in Cell, Guan and coworkers studied the changes in transcriptional rhythms in mouse livers under different diet conditions showing that opposing hepatic lipid metabolic processes become rhythmically synchronized under high-fat diet (HFD) conditions (5). They exploit this synchronization effect to optimize the treatment schedule for lipid-lowering pharmacotherapies (Figure 1).
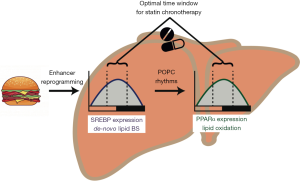
The authors used a novel deep sequencing technique, global run-on sequencing (GRO-seq) to profile daily transcriptome rhythms and rhythms of cistronic enhancer activity [through enhancer (e)RNA sequencing] in the murine liver under normal chow or 60% HFD conditions. In line with previous findings, they observed that, while most transcript rhythms became blunted under HFD conditions—indicating circadian rhythm disruption in liver physiological processes—1,343 genes became rhythmically more active under these circumstances. In line with this, eRNAs from the vicinity of these genes also became rhythmically transcribed—most of them in phase with the corresponding mRNAs—suggesting a profound reprogramming of the enhancer networks defining liver clock output in an obesogenic context.
Interestingly, lipid metabolism genes were enriched in this HFD-rhythmic fraction including genes associated with, both, de-novo fatty acid synthesis and oxidation, two counteracting processes that, under normal metabolic conditions, are confined to different phases of the circadian cycle. HFD-induced circadian eRNAs were enriched for peroxisome proliferated activated receptor (PPAR) and sterol regulatory element-binding protein (SREBP) binding DNA motifs indicating a functional role of these two transcription factor families in mediating HFD-induced liver transcriptome rhythm reprogramming. Using a virus-mediated knockdown of the SRBP adaptor protein SCAP (SREBP chaperone), they confirmed that SREBP signaling controls fatty acid synthesis rhythms under HFD conditions.
While initially the synchronization of fatty acid synthesis and breakdown was puzzling, the authors in an elegant set of experiments proceeded in showing that SREBP-mediated accumulation of fatty acids, in turn, triggers fatty acid breakdown under these conditions of energetic abundance through activation of PPARα activity, likely via 1-palmitoyl-2-oleoyl-sn-glycero-3-phosphocholine (POPC).
The acceleration of fatty acid biosynthesis and breakdown under HFD conditions was confined to the second half of the day, just before mice, as nocturnal animals, become active. The authors concluded that treatment of hyperlipidemia should, thus, be more effective when the drugs would target this critical time window. Indeed, mice treated at ZT8 (i.e., 4 h before “lights off”) with the PPARα agonist WY showed much higher responses in liver triglyceride clearance, lower blood lipid levels and stronger upregulation of lipid turnover marker genes than mice treated at ZT20 (i.e., 9 h into the dark period).
The initial finding that global dampening of circadian rhythms was accompanied by a strengthening of rhythmic transcription in some liver genes under HFD conditions was puzzling, but similar counter-intuitive findings were reported in mice with tissue-specific ablation of clock gene function (6,7). It was speculated that this may result from an unmasking effect due to uncoupling of two anti-phasic regulatory factors that, under normal conditions, yield constant output parameters (8). In the case of HFD, increased food intake during the light phase may result in an overabundance of energy in liver tissue, thus triggering simultaneous fatty acid anabolism and break-down towards the end of the rest period.
Metabolic disorders such as obesity and its sequelae are amongst the most prevalent and cost-intensive chronic diseases worldwide. In an obesogenic sedentary environment, the prevention and treatment of overweight has become one of the most pressing issues in clinical practice (9). So far, few promising pharmacological therapies have been developed and bariatric surgery—though ridden with risks and potentially dangerous side effects—remains the most effective treatment available. PPAR-targeting statins are extensively prescribed to lower blood lipid levels in obesity with the aim of preventing cardiovascular complications. It has previously been reported that PPARα expression is also rhythmic human liver (10). Moreover, some data support the idea that statin efficiency may be improved when treatment coincides with the daily maximum in cholesterol biosynthesis towards the evening (11). This paper now provides a mechanistic basis for these notions.
It is tempting to speculate that optimizing the timing of statin treatment may enhance its efficacy while reducing side effects such as muscle toxicity. It should be noted, however, that, in contrast to mice, humans are a strictly diurnal species. This would suggest that in humans, HFD-induced lipid metabolism rhythms should peak towards the end of the night. This does not coincide with the reported statin treatment optimum in the evening. Moreover, several studies suggest that timed statin treatment had little effect on the long-term therapeutic outcome (12). Such long-term effects were not studied in this paper and WY is a drug with a very short half-life of about one hour.
Nevertheless, the data by Guan and colleagues provide an important insight into the global adaptation of liver metabolism in an obesogenic metabolic environment and add to the growing body of evidence urging us to include (day-) time as a critical component in diagnosis, therapy and, potentially, prevention of metabolic diseases.
Acknowledgments
Funding: Funded by grants from the German Research Foundation (GRK-1957 & SFB-134).
Footnote
Conflicts of Interest: H Oster is a Lichtenberg fellow of the Volkswagen Foundation.
References
- Takahashi JS. Transcriptional architecture of the mammalian circadian clock. Nat Rev Genet 2017;18:164-79. [Crossref] [PubMed]
- Zhang R, Lahens NF, Ballance HI, et al. A circadian gene expression atlas in mammals: implications for biology and medicine. Proc Natl Acad Sci U S A 2014;111:16219-24. [Crossref] [PubMed]
- Lemmer B, Oster H. The Role of Circadian Rhythms in the Hypertension of Diabetes Mellitus and the Metabolic Syndrome. Curr Hypertens Rep 2018;20:43. [Crossref] [PubMed]
- Kohsaka A, Laposky AD, Ramsey KM, et al. High-fat diet disrupts behavioral and molecular circadian rhythms in mice. Cell Metab 2007;6:414-21. [Crossref] [PubMed]
- Guan D, Xiong Y, Borck PC, et al. Diet-Induced Circadian Enhancer Remodeling Synchronizes Opposing Hepatic Lipid Metabolic Processes. Cell 2018;174:831-842.e12. [Crossref] [PubMed]
- Kolbe I, Husse J, Salinas G, et al. The SCN Clock Governs Circadian Transcription Rhythms in Murine Epididymal White Adipose Tissue. J Biol Rhythms 2016;31:577-87. [Crossref] [PubMed]
- Chaix A, Zarrinpar A, Miu P, et al. Time-restricted feeding is a preventative and therapeutic intervention against diverse nutritional challenges. Cell Metab 2014;20:991-1005. [Crossref] [PubMed]
- Lamia KA, Storch KF, Weitz CJ. Physiological significance of a peripheral tissue circadian clock. Proc Natl Acad Sci U S A 2008;105:15172-7. [Crossref] [PubMed]
- Heymsfield SB, Wadden TA. Mechanisms, Pathophysiology, and Management of Obesity. N Engl J Med 2017;376:254-66. [Crossref] [PubMed]
- Anafi RC, Francey LJ, Hogenesch JB, et al. CYCLOPS reveals human transcriptional rhythms in health and disease. Proc Natl Acad Sci U S A 2017;114:5312-7. [Crossref] [PubMed]
- Saito Y, Yoshida S, Nakaya N, et al. Comparison between morning and evening doses of simvastatin in hyperlipidemic subjects. A double-blind comparative study. Arterioscler Thromb 1991;11:816-26. [Crossref] [PubMed]
- Izquierdo-Palomares JM, Fernandez-Tabera JM, Plana MN, et al. Chronotherapy versus conventional statins therapy for the treatment of hyperlipidaemia. Cochrane Database Syst Rev 2016;11:CD009462. [PubMed]