Effect of tomato extract supplementation against high-fat diet-induced hepatic lesions
Introduction
Carotenoids, isoprenoid compounds involved in a myriad of natural functions (1) continue to attract much interest due to accumulating evidence indicating that some of them—their metabolites and/or related compounds are involved in different biologic functions in the human body and provide diverse health benefits. More specifically, the health benefits of lycopene (LY, ψ,ψ-carotene, Figure 1), the red pigment found in some plant foods (tomatoes, watermelon, grapefruit, and other fruits) and of its oxidative metabolites have been the subject of major studies in the last decade (2).
Evaluating the health benefits of carotenoids in general and LY, in particular, to study the effects of pure compounds vs. extracts containing other potential health-promoting agents is of interest. In this regard, tomatoes—most tomato varieties commonly used for human consumption other than LY, are also good sources of the colorless carotenoids phytoene (PT, 7,8,11,12,7',8',11',12'-octahydro-ψ,ψ-carotene, Figure 1) phytofluene (PTF, 7,8,11,12,7',8'-hexahydro-ψ,ψ-carotene, Figure 1), and other micronutrients such as vitamin C, E and flavonoids (3-5). PT is formed from the condensation of two molecules of geranylgeranyl pyrophosphate. This reaction is catalyzed by the enzyme phytoene synthase is the first step in the biosynthesis of carotenoids (6). PTF, with 5 conjugated double bonds, is formed by desaturation from PT; a reaction catalyzed in plants by the enzyme phytoene desaturase (6). Notwithstanding, the biological effects of PT and PTF have not been as extensively studied as those of other carotenoids. Both carotenoids have been reported to be bioavailable and accumulate in several tissues of both rats (5,7,8) and humans (9,10). Likewise, they have been reported to inhibit LDL oxidation in vitro (11) and to enhance the protection of coenzyme Q10 against skin inflammation (12). Interestingly, evidence has been provided that tomato-based extracts containing LY, PT and PTH have a greater protective effect than LY alone against UV-light induced erythema (13).
In one of the earliest studies on the relationships between carotenoids and the prevention of carcinogenesis, PT seemed to reduce the number of UV-B induced skin tumors in mouse in relation to the controls (13). It has been shown that PTF inhibited the growth of HL-60 human promyelocytic leukemia cells after 5 days of treatment by some 23% (14) and LY, PT, PTF and β-carotene can inhibit the cancer cell proliferation induced by some estrogens, which are the most important risk factors in mammary and endometrial cancers (15). Recently, we demonstrated that tomato extract, as compared with LY displayed more efficacy in protecting against high fat diet (HFD)-induced liver inflammation by decreasing both the number of inflammatory foci and the expression of multiple proinflammatory cytokines (16). Considering the accumulating evidence that PT and PTF can be bioactive compounds (5) and in connection with other experiments (16,17), in this study we examined PT, PTF, LY, lipid profiles and cholesterol in rats fed either control diet (CD) or HFD with or without TE supplementation in relation to high-fat-diet related hepatic inflammation and lipid profiles, and carcinogenesis.
Materials and methods
Animals, diets and groups
The maintenance and husbandry of rats was described elsewhere (18). Briefly, eight-week old male Sprague-Dawley rats (Charles River Co., Wilmington, MA) were fed either a Lieber-DeCarli control diet (CD, 35% energy from fat) or HFD (71% energy from fat) (Dyets Inc., Bethlehem, PA) ad libitum for 6 weeks. All rats were randomly assigned to 4 groups (n=8): (I) CD; (II) CD+TE; (III) HFD and (IV) HFD+TE. Dietary intake was monitored daily and body weight was measured weekly. At the end of this experiment, plasma was collected by cardiac puncture while the liver was excised and frozen in liquid nitrogen and stored at –80 °C. The Institutional Animal Care and Use Committee at the USDA Human Nutrition Research Center on Aging approved the animal protocol.
Dietary supplements
The TE was kindly provided by LycoRed Ltd. (Beer-Sheva, Israel). According to the manufacturer it contained ~6% LY, ~1.5% natural tocopherols, ~1% PT & PTF and ~0.2% beta-carotene. The dosage of TE (250 mg TE/kg BW per day) was chosen to contain an equivalent amount of LY to that from pure LY used (15 mg LY/kg BW per day). The TE supplements for each group were weighed and homogenized with liquid diet that was shown to be sufficiently consumed by rats from our previous studies. The diets were prepared twice a week and were stored at –4 °C in opaque bottles to prevent degradation of the carotenoids.
HPLC analysis
One hundred mg of liver tissue or 1 mL of plasma were homogenized in 2-4 mL of a mixture of saline and ethanol (1:2, v/v). Subsequently, 5 mL of a mixture of hexane and ether (1:1, v/v) were added and vortexed for 1 min. After centrifugation (2,000 g ×10 min at 4 °C) the epiphase was collected and the hypophase was re-extracted once more in the same conditions. The two epiphases were pooled and dried out under a stream of nitrogen gas and eventually re-dissolved in 100 µL of ethanol and ether (2:1, v/v). A 50-µL sample of the final extract was injected into the HPLC system. All the operations were carried out under dim red light. The HPLC system consisted of a 2,695 Alliance separation module fitted with a 2,996 photodiode array detector (Waters Corporate, Milford, MA). The separations were carried out on a YMC C30 column (S-3 micron, 4.6 mm × 150 mm) (YMC, Wilmington, NC, USA). Methanol (MeOH) and methyl-tert-butyl ether (MTBE) were used in the mobile phase according to the following gradient: 0 min, 90% A and 10% B; 20 min, 85% A and 15% B; 30 min, 40% A and 60% B; 37 min, 40% A and 60% B; 45 min, 90% A and 10% B; 50 min, 90% A and 10% B. The flow was 1 mL/min. PT, PTF and LY were quantified at 285, 348 and 472 nm, respectively with dose-response curves made with appropriate standards. The PT standard was obtained from oil containing that pigment at a concentration of 3.4% (w/w) by injecting an extract and collecting the fraction corresponding to that pigment. The PTF standard was obtained likewise from a tomato extract rich in that pigment. The oil and the TE were kindly provided by Vitan Ltd. (Kievskaya, Ukraine) and LycoRed Ltd. (Beer-Sheva, Israel), respectively, whilst the LY standard was a gift from BASF (Ludwigshafen, Germany).
Histological examination of inflammatory foci and immunohistochemical assay
Formalin-fixed and paraffin-embedded liver tissues were processed routinely for hematoxylin and eosin (H&E) staining. The numbers of hepatic lobular inflammatory foci were counted at 20× magnification from 10 randomly selected fields under light microscope by two independent investigators, as previously described. The mean number of the foci for each group was determined and then compared. AHF expressing P-GST were detected and analyzed by immunohistochemical assay, as described previously (16,19).
Biochemical assay for lipids
Lipids were extracted using chloroform: methanol (2:1, v/v) mixture according to routine procedures. Commercial available kits were then used to measure total triglyceride (TG) (Cat. No: 10010303, Cayman Chemical Inc, MI), total cholesterol (TC) (Cat. No: TE289, Wako Chemical Inc, VA) and free fatty acids (FFA) (Cat. No: K612-100, Biovision Inc, CA).
Gene expression by real-time PCR
Total RNA was isolated from the liver by TriPure Isolation Reagent (Roche Diagnostics, Indianapolis, IN) according to the instructions. cDNA was then prepared from the RNA samples using M-MLV reverse transcriptase (Invitrogen, Carlsbad, CA) and an automated thermal cycler (Bio-Rad Laboratories, Hercules, CA). After quantification and qualification, PCR reactions for the amplification of cDNAs of interests were carried out using a 20 µL reaction mixture containing 10 µL 2X SYBR Green Supermix, 0.4 µL of 10 µmol/L primer mix (including forward and reverse primers) and 2.5 µL cDNA diluted in RNase-free water. Cycling conditions were 50 °C for 2 min and 95 °C for 10 min, followed by 40 cycles at 95 °C for 15 sec and 60 °C for 1 min. Gene-specific primer sequences were designed using the Primer Express version 2.0 software (Applied Biosystems, Foster City, CA). PCR results were then normalized to the levels of glyceraldehyde-3-phosphate dehydrogenase (GAPDH) and calculated by reference to the average value for the control group using the comparative Ct method. For each sample and each gene, PCR reactions were carried out in duplicate and repeated twice. The primer pairs used for real-time PCR analysis are described in Table 1.
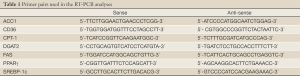
Full Table
Statistical analysis
One-way ANOVA followed by Turkey post-hoc analysis was performed. All variables were expressed as means ± SEM (standard error). A difference was considered to be significant at P<0.05.
Results
Assessment of body/liver weights, hepatic inflammation and carcinogenesis
Body weight gain and liver weight showed no significant changes among all four groups (Table 2). HFD feeding resulted in a remarkable higher inflammatory cell infiltrates in the liver, as compared with CD (Figure 2, Table 2). This HFD-induced inflammatory foci was significantly reduced by supplementation of TE (e.g., more than 40%, Table 2). No apparent changes were observed among all CD-fed groups. The incidence of P-GST positive AHF did not differ among the four groups (data not shown). The multiplicity or the average number of AHF was significantly inhibited up to 80% by dietary supplementations of TE as compared with that of HFD alone (P<0.01).
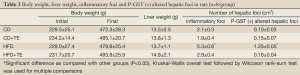
Full Table
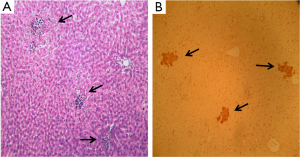
Plasma and hepatic carotenoid levels
The ratio LY:PT:PTF in the TE was 1.0:0.6:0.3, and whilst the levels of LY and PT corresponded mainly to a major geometrical isomer, those of PTF corresponded to 5 of them (Figure 3). No carotenoids were detected in both CD and HFD groups without TE supplementation (data not shown). Interestingly, LY was not the major carotenoid in plasma as we expected (Figure 4). The levels of LY were the lowest in both CD and HFD rats. Independently of the amount of fat provided in the diet. PT was the major plasma carotenoid found at the end of the study followed by PTF. More specifically, the plasma levels of PT and PTF were approximately 7-fold and 3.5-fold higher, respectively, than those of LY in rats that received CD plus TE supplementation. In the case of HFD plus TE supplementation, the levels of PT and PTF were much higher relative to those of LY, specifically 34-fold and 9-fold, respectively. Concerning the hepatic levels of carotenoids at the end of the intervention in both groups, the levels of LY were the lowest whereas those of PT were the highest. In this case, the levels of both PTF and LY in the rats receiving the HFD were higher, the differences in the case of LY being significant (P<0.05) (Figure 4).
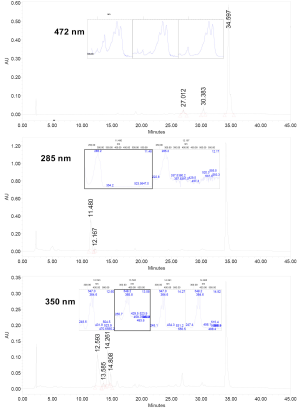
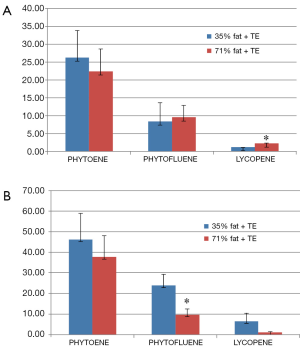
Plasma and hepatic lipid levels
Neither the amount of fat in the diet nor the supplementation with the tomato extract led to significant changes in the plasma levels of triglycerides (TG) and frees fatty acids (FFA). The increase of fat in the diet led to significant higher levels of total cholesterol (TC) in the HFD group relative to the CD group. Interestingly, the supplementation with TE led to a significant decrease in the plasma levels of TC in both control and high-fat diet groups (Table 3). The supplementation with the TE led to a significant (P<0.05) increase in the hepatic levels of all the lipids assessed with the only exception of those of FFA in rats receiving control diets. As expected, the hepatic levels of lipids in the HFD group were significantly higher than those found in the CD group (Table 3).
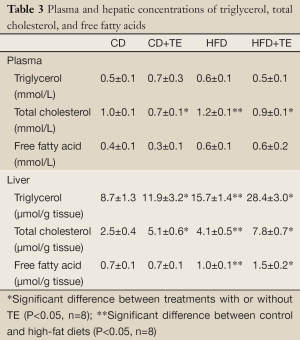
Full Table
Changes in the mRNA levels of genes related to lipid metabolism
Cluster of differentiation 36 (CD36) is a type of class B scavenger receptor widely expressed (hepatocytes, vascular endothelium, adipocytes, skeletal muscle, dendritic cells, epithelia of the retina, breast, and intestine, etc.) have a high affinity for native and modified lipoproteins (20,21). This transmembrane protein is currently being the subject of research in relation to their role in the bioavailability of carotenoids (22,23). We have found that the gene expression of hepatic CD36, one of the major factors responsible for plasma FFA uptake by the liver, increased from CD to HFD, and that independently of the diet, the TE supplementation led to significant increases in their mRNA levels (Figure 5).
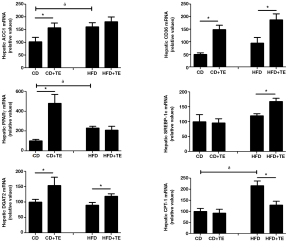
As the essential rate-limiting enzymes involved in hepatic lipogenesis and TG synthesis, the mRNA levels of acetyl-CoA carboxylase (ACC)-1 were significantly increased in the rats receiving CD plus TE relative to those receiving CD. However, the supplementations with TE did not lead to significant changes in the HFD-fed rats (Figure 5). In any case, mRNA levels of (ACC)-1 were significant higher in rats receiving HFD relative to those receiving CD (Figure 5). These observations seem to indicate that the TE favor the hepatic lipogenesis in rats receiving normal diets but not in those receiving diets with a high fat content.
Diacylglycerol acyltransferase (DGAT) catalyzes the conversion of diacylglycerols into triacylglycerols, the final step in the biosynthesis of the latter compounds. There are several isoforms of the enzyme with different catalytic properties, tissue distribution, subcellular localization, and physiological functions. In our model, the supplementation with TE led to significant increases in the mRNA (DGAT)-2 levels in both CD and HFD-fed rats (Figure 5). The amount of fat in the diet of rats not receiving supplementation did not significantly affect its mRNA levels.
Carnitine palmitoyltransferase (CPT)-1 is an enzyme with an essential role in the oxidation of fatty acids in the mitochondria of mammalian tissues. In our rat model, we observed that the supplementation with TE did not affect significantly the mRNA levels of this enzyme in the CD group, although it did decrease them in the HFD-group. Likewise, a significant induction of the transcription of the (CPT)-1 gene in HFD rats relative to CD rats was observed (Figure 5).
The peroxisome proliferator-activated receptors (PPARs) located in the nuclei play key roles in lipid metabolism, cellular differentiation and inflammation and other processes. Three PPAR subtypes (α, γ and δ) with different expression patterns and functions have been described. The most intensively studied isoform is PPARγ, which is expressed predominantly in the intestine and adipose tissue, among other functions, promotes lipid storage. The sterol-regulatory element binding proteins (SREBP) are membrane-bound transcription factors that regulate lipogenic and glycolytic genes, including key genes involved in the metabolism of cholesterol. Three members of the SREBP family have been described in mammals, namely SREBP-1a, -1c and -2. In our study, the levels of PPARγ mRNA were significantly higher in the rats receiving HFD relative to the control, whilst the TE supplementation led to a significant increase of the transcript only in the case of the CD+TE group. In contrast, the amount of fat in the groups not receiving TE did not affect significantly the transcription of SREBP-1, whilst the supplementation led to an increase of the mRNA levels of this transcription factor only in rats receiving HFD (Figure 5).
Discussion
The present study revealed a clear partition between increased hepatic lipid accumulation and decreased liver inflammation and preneoplastic foci by dietary supplementation of TE. However, the underlying mechanism remains poorly understood. At first glance, our observations of lipid accumulation might be seen as harmful. Since accumulation of triglycerides in hepatocytes is the hallmark of nonalcoholic fatty liver disease (NAFLD). However, this fact should be a compensatory mechanism of important pathophysiological relevance since triglyceride synthesis might be able to protect hepatocytes from lipotoxicity by buffering the accumulation of FFA, and detoxify excess intracellular fatty acid (24). Free fatty acids might cause liver damage by several mechanisms through increased ROS production by upregulation of microsomal enzymes (such as Cytochrome P4502E1) (25) and lipid peroxidation reaction from oxidation excessive (26). Results from our previous study in this model showed that hepatic lipid peroxidation and CYP2E1 protein were significantly reduced by TE (16). In this study, TE supplementation inhibited inflammatory responses and downregulated CPT-1 levels; a rate-limiting enzyme involved in the transport of long-chain fatty acids into mitochondrial matrix. To the best of our knowledge, this is the first piece of evidence showing that dietary TE could have a positive effect on both hepatic protection against inflammation and hepatic lipid accumulation. In addition, it seems that there is a positive effect on TE’s potential regulation on transcriptional factors involved in lipid metabolism in the liver. However, the long-term effects on fat accumulation in the liver by tomato extract or its components is worthy of our attention, especially considering the close association between obesity, steatosis and insulin resistance. The investigation of TE and its carotenoid components on metabolic syndrome and hepatic insulin sensitivity is warranted in future studies. In vitro systems could also be used as a tool to specifically investigate the potential effects of LY, PT or PE on the insulin signaling pathway and its regulation on cellular utilization of glucose and fatty acids.
One of our more intriguing findings of this study is the significant upregulation, instead of downregulation, of almost all hepatic lipid profiles by dietary TE supplementation in either CD- or HFD-fed rats. Upregulation of hepatic lipid profiles seems to be diet-independent and through different mechanisms. In CD-fed rats, supplementation of TE led to increased FFA uptake, lipogenesis and TG synthesis by its upregulation on hepatic CD36, ACC1 and DGAT2. On the other hand, in HFD-fed rats, CD36 was increased, while DGAT2 was significantly lower than CPT-1 suggesting that altered lipid oxidation could also be involved in this process. The upregulation of lipid influx in CD-fed rats could also be affected by increased PPARγ, which was significantly induced in CD plus TE vs. CD and has been indicated to be an essential transcriptional factor in hepatocytes to enhance both lipid synthesis and accumulation leading to steatosis. However, SREBP-1c, another key transcriptional factor regulating lipogenesis was found highly induced in the HFD plus TE but not in the CD plus TE group. More studies need to be conducted to corroborate our findings to ensure whether this effect is only specific to different fat diet, animal species or tomato carotenoid dosage.
In our study we found that TE supplementation results in a significantly higher accumulation of both PT and PTF than LY in livers of rats. The accumulation of the colorless carotenoids PF and PTF in plasma and different tissues of rats have also been evaluated by other authors [reviewed by (5)]. It has been reported that both the (all-E)- and the (9Z)-phytoene isomers from a Dunaliella bardawil dried powder (50 g, to make up 1 g/kg diet, 2 wk of supplementation) were bioavailable in female rats. High levels of PF were found in liver, and lower ones in plasma and adrenal, brain, heart, kidney, lung, and spleen tissues. Interestingly, the original isomers ratio found in the algal diet [1:1] was found in plasma and adrenal, while in the liver, spleen and kidney the ratio was reduced to 1:3; the (all-E)-phytoene isomer accumulating preferentially (7). In another study, male rats were fed a powder diet containing PT, PTF and LY (0.015, 0.012, and 0.011 g/kg diet, respectively). After 30-day, the hepatic levels of PTF were some 1.5-fold higher than those of PF or LY. In contrast, LY and PF are the major carotenoids in prostate lobes and seminal vesicles. When tomato powder-fed rats received a single oral dose of approximately 2.7 mg of each colorless carotenoid, the percentages of increase of TPF were greater than that of PT in liver, serum, and adipose tissues (8). Taken together, our data seems to indicate that there are differences in the metabolism of tomato carotenoids both in CD and HFD rats. With levels of carotenoids in plasma and tissues dependent upon manifold factors related to their absorption (e.g., release from the matrix, incorporation into micelles, among many others) and usage once absorbed (e.g., stability towards electrophiles and enzymatic cleavage, among others). It seems important to understand the chemical differences between absorption and usage once absorpbed could be related to their different metabolic fates. On the one hand, it is interesting to note that whilst the main LY isomer in tomatoes is the (all-E)-isomer, the main PT isomer in tomato and carotenogenic organisms in general is the (15Z)-isomer (6). Likewise, different isomers of PTF have been found to be used in this study, as stated before (Figure 1). This fact is important as there are studies that seem to indicate that in some cases Z-isomers are better micellarized or more bioavailable than their (all-E)-counterparts (27-30). It has to be taken into account that the “kinked” Z-isomers are shorter than the linear (all-E)-isomers and are less prone to aggregation, which can have an impact in their solubility, bioavailability, sub-cellular location and organization and function [reviewed in (4,31-34)]. Different geometrical isomers of carotenoids seem to behave differently when exposed to oxidants (35) and could be preferentially cleaved by carotenoid monooxygenases (36), which are known to be expressed in different tissues of mammals like liver, intestinal tract, kidney, prostate, lung and testis, among others [reviewed in (33)]. In addition, PT, PTF and LY differ in the number of conjugated double bonds in their molecules (3, 5 and 11, respectively). This has implications in their chemical reactivity and therefore their stability. For instance, it is accepted that all carotenoids react easily with electrophiles, although their relative reactivity is dependent on the length of their chromophore and the nature of their end groups (31). Future studies on the different behaviour of these tomato carotenoids concerning isomerization and oxidation will shed some light on these aspects. Interestingly, we have found that TE upregulates the transcription of the CD36 receptor which is involved in the intake of lipoproteins by the hepatocyte. Since carotenoids circulate in lipoproteins, the increase in the expression of this receptor could have an effect on the hepatic levels of carotenoids. The levels of both PTF and LY were higher in the HFD plus TE group than in the CD plus TE group and that this correlates well with the pattern of the transcription of the CD36 gene. Lower circulating levels of PTF and LY did not lead to lower hepatic concentration, supporting the possibility that a concentration-independent mechanism could be involved in both PTF and LY’s uptake by the hepatocytes or their hepatic metabolism is affected as a result of an increase of fat in their diet.
The data concerning the transcription of key enzymes involved in the lipid metabolism points to the fact that the amount of fat in the diet of unsupplemented rats favors the lipogenesis and the oxidation of fatty acids in liver but does not have a significant effect in the synthesis of triaceylglycerols. The effects of the TE supplementation is only consistent in the case of DGAT and seems to favor the synthesis of triaceylglycerols independently of the type of diet received. In contrast, the supplementation favors the lipogenesis only in rats receiving CD and the mitochondrial oxidation of fatty acids in the group receiving HFD. Overall, it can be concluded that the carotenoid-rich TE not only inhibits HFD induced inflammation and promotes hepatic carcinogenesis, but also affects the transcription of enzymes involved in the lipid metabolism differently as a function of the amount of fat provided in the diet. Furthermore, it appears that TE has a role in the transcriptional regulation by PPARγ and SREBP-1c of the expression of genes related to the lipid metabolism both in rats receiving CD and HFD.
Acknowledgements
This work was a joint research program Supported by NIH grant R01CA104932; USDA grant 1950-51000-064S; A.J. Melendez-Martinez acknowledges funding through the Spanish programs Ramón y Cajal and the National Program for the Mobility of Human Resources, refs. RYC-2010-07115 and JC-2009-00176. The assistance of Connie Hu and Jun-Goo Kwak is gratefully acknowledged.
Disclosure: The authors declare no conflict of interest.
References
- Britton G, Liaaen-Jensen S, Pfander H. eds. Carotenoids, vol. 5: Nutrition and Health. Boston: Ma Birkhäuser Verlag, 2009.
- Wang XD. Lycopene metabolism and its biological significance. Am J Clin Nutr 2012;96:1214S-22S. [PubMed]
- Khachik F, de Moura FF, Zhao DY, et al. Transformations of selected carotenoids in plasma, liver, and ocular tissues of humans and in nonprimate animal models. Invest Ophthalmol Vis Sci 2002;43:3383-92. [PubMed]
- Meléndez-Martínez AJ, Fraser PD, Bramley PM. Accumulation of health promoting phytochemicals in wild relatives of tomato and their contribution to in vitro antioxidant activity. Phytochemistry 2010;71:1104-14. [PubMed]
- Engelmann NJ, Clinton SK, Erdman JW Jr. Nutritional aspects of phytoene and phytofluene, carotenoid precursors to lycopene. Adv Nutr 2011;2:51-61. [PubMed]
- Fraser PD, Bramley PM. The biosynthesis and nutritional uses of carotenoids. Prog Lipid Res 2004;43:228-65. [PubMed]
- Werman MJ, Mokady S, Ben-Amotz A. Bioavailability of the isomer mixture of phytoene and phytofluene-rich alga Dunaliella bardawil in rat plasma and tissues. J Nutr Biochem 2002;13:585-91. [PubMed]
- Campbell JK, Engelmann NJ, Lila MA, et al. Phytoene, phytofluene, and lycopene from tomato powder differentially accumulate in tissues of male fisher 344 rats. Nutr Res 2007;27:794-801. [PubMed]
- Khachik F, Carvalho L, Bernstein PS, et al. Chemistry, distribution, and metabolism of tomato carotenoids and their impact on human health. Exp Biol Med (Maywood) 2002;227:845-51. [PubMed]
- Porrini M, Riso P, Brusamolino A, et al. Daily intake of a formulated tomato drink affects carotenoid plasma and lymphocyte concentrations and improves cellular antioxidant protection. Br J Nutr 2005;93:93-9. [PubMed]
- Shaish A, Harari A, Kamari Y, et al. A carotenoid algal preparation containing phytoene and phytofluene inhibited LDL oxidation in vitro. Plant Foods Hum Nutr 2008;63:83-6. [PubMed]
- Fuller B, Smith D, Howerton A, et al. Anti-inflammatory effects of CoQ10 and colorless carotenoids. J Cosmet Dermatol 2006;5:30-8. [PubMed]
- Aust O, Stahl W, Sies H, et al. Supplementation with tomato-based products increases lycopene, phytofluene, and phytoene levels in human serum and protects against UV-light-induced erythema. Int J Vitam Nutr Res 2005;75:54-60. [PubMed]
- Nara E, Hayashi H, Kotake M, et al. Acyclic carotenoids and their oxidation mixtures inhibit the growth of HL-60 human promyelocytic leukemia cells. Nutr Cancer 2001;39:273-83. [PubMed]
- Hirsch K, Atzmon A, Danilenko M, et al. Lycopene and other carotenoids inhibit estrogenic activity of 17beta-estradiol and genistein in cancer cells. Breast Cancer Res Treat 2007;104:221-30. [PubMed]
- Wang Y, Ausman LM, Greenberg AS, et al. Dietary lycopene and tomato extract supplementations inhibit nonalcoholic steatohepatitis-promoted hepatocarcinogenesis in rats. Int J Cancer 2010;126:1788-96. [PubMed]
- Sharoni Y, Linnewiel-Hermoni K, Khanin M, et al. Carotenoids and apocarotenoids in cellular signaling related to cancer: a review. Mol Nutr Food Res 2012;56:259-69. [PubMed]
- Wang Y, Ausman LM, Greenberg AS, et al. Nonalcoholic steatohepatitis induced by a high-fat diet promotes diethylnitrosamine-initiated early hepatocarcinogenesis in rats. Int J Cancer 2009;124:540-6. [PubMed]
- Ye Q, Lian F, Chavez PR, et al. Cytochrome P450 2E1 inhibition prevents hepatic carcinogenesis induced by diethylnitrosamine in alcohol-fed rats. Hepatobiliary Surg Nutr 2012;1:5-18. [PubMed]
- Calvo D, Gómez-Coronado D, Suárez Y, et al. Human CD36 is a high affinity receptor for the native lipoproteins HDL, LDL, and VLDL. J Lipid Res 1998;39:777-88. [PubMed]
- Febbraio M, Hajjar DP, Silverstein RL. CD36: a class B scavenger receptor involved in angiogenesis, atherosclerosis, inflammation, and lipid metabolism. J Clin Invest 2001;108:785-91. [PubMed]
- Moussa M, Gouranton E, Gleize B, et al. CD36 is involved in lycopene and lutein uptake by adipocytes and adipose tissue cultures. Mol Nutr Food Res 2011;55:578-84. [PubMed]
- Borel P, de Edelenyi FS, Vincent-Baudry S, et al. Genetic variants in BCMO1 and CD36 are associated with plasma lutein concentrations and macular pigment optical density in humans. Ann Med 2011;43:47-59. [PubMed]
- Yamaguchi K, Yang L, McCall S, et al. Inhibiting triglyceride synthesis improves hepatic steatosis but exacerbates liver damage and fibrosis in obese mice with nonalcoholic steatohepatitis. Hepatology 2007;45:1366-74. [PubMed]
- Leclercq IA, Farrell GC, Field J, et al. CYP2E1 and CYP4A as microsomal catalysts of lipid peroxides in murine nonalcoholic steatohepatitis. J Clin Invest 2000;105:1067-75. [PubMed]
- Penumetcha M, Khan N, Parthasarathy S. Dietary oxidized fatty acids: an atherogenic risk? J Lipid Res 2000;41:1473-80. [PubMed]
- Failla ML, Chitchumroonchokchai C, Ishida BK. In vitro micellarization and intestinal cell uptake of cis isomers of lycopene exceed those of all-trans lycopene. J Nutr 2008;138:482-6. [PubMed]
- Unlu NZ, Bohn T, Francis DM, et al. Lycopene from heat-induced cis-isomer-rich tomato sauce is more bioavailable than from all-trans-rich tomato sauce in human subjects. Br J Nutr 2007;98:140-6. [PubMed]
- Boileau AC, Merchen NR, Wasson K, et al. Cis-lycopene is more bioavailable than trans-lycopene in vitro and in vivo in lymph-cannulated ferrets. J Nutr 1999;129:1176-81. [PubMed]
- Ferruzzi MG, Lumpkin JL, Schwartz SJ, et al. Digestive Stability, micellarization, and uptake of beta-carotene isomers by Caco-2 human intestinal cells. J Agric Food Chem 2006;54:2780-5. [PubMed]
- Britton G. Structure and properties of carotenoids in relation to function. FASEB J 1995;9:1551-8. [PubMed]
- Schieber A, Carle R. Occurrence of carotenoid cis-isomers in food: Technological, analytical, and nutritional implications. Trends Food Sci Tech 2005;16:416-22.
- Wang XD. Biological activities of carotenoid metabolites. In: Britton G, Liaaen-Jensen S, Pfander H. eds. Carotenoids Volume 5: Nutrition and Health. Basel: Birkhauser, 2009:383-408.
- Canene-Adams K, Erdman JW Jr. Absorption, transport, distribution in tissues and bioavailability. In: Britton G, Liaaen-Jensen S, Pfander H. eds. Carotenoids Volume 5: Nutrition and Health. Basel: Borkhauser, 2009:115-48.
- Böhm V, Puspitasari-Nienaber NL, Ferruzzi MG, et al. Trolox equivalent antioxidant capacity of different geometrical isomers of alpha-carotene, beta-carotene, lycopene, and zeaxanthin. J Agric Food Chem 2002;50:221-6. [PubMed]
- Hu KQ, Liu C, Ernst H, et al. The biochemical characterization of ferret carotene-9',10'-monooxygenase catalyzing cleavage of carotenoids in vitro and in vivo. J Biol Chem 2006;281:19327-38. [PubMed]