Inhibition of diethylnitrosamine-initiated alcohol-promoted hepatic inflammation and precancerous lesions by flavonoid luteolin is associated with increased sirtuin 1 activity in mice
Introduction
Liver cancer is the third leading fatal cancer in the world (1). Chronic and excessive alcohol consumption is a major risk factor for liver cancer in the United States and it has been estimated that 32-45% of all cases are due to alcohol abuse (2). Alcohol readily diffuses across membranes and distributes through all cells and interacts with proteins and cell membranes (3), disrupts fatty acid oxidation, thereby contributing to steatosis onset and liver injury (4). We and others have shown that alcohol has a promoter action on the development of chemically induced hepatic cancer (2,5,6). Although the molecular mechanisms of alcohol induced carcinogenesis are not fully understood, increasing evidence suggests a link between alcohol metabolism and cancer (7-10). Alcohol metabolism involves the enzymes aldehyde dehydrogenase (ALDH), alcohol dehydrogenase (ADH), cytochrome P4502E1 (CYP2E1), and catalase, generating acetaldehyde and reactive oxygen species (ROS) (3). Acetaldehyde adducts and ROS can activate the adaptive immune response and the production of proinflammatory cytokines (4,11,12), leading to inflammation of the liver (7,13). Indeed, cellular and molecular abnormalities due to inflammatory condition might represent an early step of hepatocarcinogenesis and is considered a key factor in the development of cancer (11,14).
Sirtuin 1 (SIRT1), the mammalian ortholog of yeast sirtuin silent information regulator 2, is a conserved NAD+-dependent protein deacetylase expressed in various tissues including the liver (15). It has been implicated in various biological processes, and its expression and activity in the liver can be altered by alcohol intake (16,17). SIRT1 activation has been described as a protective factor in many diseases (18-20), including high fat diet promoted hepatocarcinogenesis (15,21). Despite this evidence, the role of SIRT1 and liver cancer is controversial, reported both as a tumor promoter and as a tumor suppressor protein (22-24). SIRT1 has also been a target for dietary intervention in many models, including liver injury (23), addressing questions to whether dietary compounds can modulate SIRT1 expression and/or activity and exhibit anti-carcinogenic effects.
Epidemiological studies have shown that a diet rich in plant-derived foods is consistently associated with reduced risk of developing chronic diseases, supporting recommendations to increase the consumption of plant-derived foods (25,26). Although it is not clear which compounds in plant foods are responsible for this preventive effect, evidence suggests that flavonoids may participate in this activity (26,27). Luteolin is one of the most common flavonoids present in edible plants, with celery, parsley, broccoli, peppers, cabbages, and herbal spices as the main food sources of luteolin (28). It has been shown that luteolin exhibits anti-inflammatory and anticancer properties by inhibiting pro inflammatory cytokines, inducing apoptosis and preventing DNA damage and carcinogen metabolic activation (25,29-31), but most of the studies were conducted in vitro. One previous study described anti-carcinogenic potential of luteolin against hepatocellular carcinoma in rats (32). So far to our knowledge, there is no in vivo study addressing whether luteolin protection against alcohol induced hepatic carcinogenesis.
The present study investigated a dietary intervention with luteolin at 30 mg/kg of body weight for 21 days in DEN-initiated ethanol-promoted hepatic carcinogenesis in mice. The presence of steatosis, inflammation, and preneoplastic lesions and the involvement of SIRT1 pathway and CYP2E1 in this model were examined.
Methods
Study design
The experimental protocol was adapted from a well-established animal model to study liver carcinogenesis (6,33-35). All animal protocols were approved by the Institutional Animal Care and Use Committee at the Jean Mayer-USDA Human Nutrition Research Center on Aging at Tufts University. Male C57BL6 mice were distributed into four groups by weight-matching, according to diet and treatment. Diethylnitrosamine (DEN, Sigma Aldrich, St. Louis, MO; i.p. 25 mg/kg of BW) was injected into two DEN treated groups at 2 weeks of age. After an adaptation period, all mice were group pair-fed with Lieber-DeCarli (Dyets Inc., Bethlehem, PA, USA) liquid control diet or alcoholic diet (EtOH diet, 27% total energy from ethanol) and supplemented with a dose of 30 mg luteolin/kg BW per dayfor a treatment period of 21 days. In the control diet, ethanol was replaced by an isocaloric amount of maltodextrin (Purina Test diets, Richmond, IN, USA). Therefore, the animal groups were: (I) control group (no DEN injection or ethanol feeding; (II) control + DEN (DEN injected, no ethanol feeding); (III) DEN + EtOH (DEN injected and ethanol feeding); (IV) DEN + EtOH + L (DEN injected and ethanol feeding + luteolin treatment). After the treatment period, all mice were terminally exsanguinated under anesthesia and liver tissue was collected and frozen for analysis. The study design is presented in Figure 1.
Luteolin supplementation
Luteolin commercial compound was purchased from Sigma Aldrich (St. Louis, MO, USA), and was incorporated directly into the diet to achieve a homogenous diet mixture. The rationale for luteolin dose of 30 mg/kg of body weight was based on previous studies reporting an anti-carcinogenic action of luteolin (36,37), daily human intake of luteolin, and data about luteolin absorption in rodents and humans that demonstrated that luteolin has similar pharmacokinetics in the two species (38,39). Luteolin intake in the American population is relatively low, varying from 0.01 to 8.88 mg/d, with an average of 0.1 mg/d (27,40,41). Using an established equation to calculate the dosage equivalence for human consumption (42,43), the dose of 30 mg/kg of body weight in mice is equivalent to 0.081 mg/kg of body weight for humans, meaning a dose within the physiologic range.
Histopathological evaluation
5 µm sections of formalin-fixed, paraffin-embedded liver tissue were stained with H&E. Liver histopathology was graded according to steatosis magnitude (both macro- and micro-vesicular fat accumulation), and the degree of liver inflammation severity as described previously (15,44). Briefly, the degree of steatosis was graded 0-4 (grading 0≤5%, 1=5-25%, 2=26-50%, 3=51-75%, 4>75%), based on the average percent of fat-accumulated hepatocytes per field at 100× magnification under H&E staining in 20 random fields. Inflammatory foci which mainly constitute mononuclear inflammatory cells were evaluated by the number of inflammatory-cell clusters in 20 random fields at 100× magnification, as reported as number of inflammatory foci per cm2. Preneoplastic lesions were evaluated and presented by the incidence and number of basophilic and eosinophilic foci per cm2 in 20 random fields at 100× magnification (45).
Protein isolation and western blotting
Liver sections were lysed in cold whole cell lysis buffer (25 mM Hepes at Ph =7.5, 300 mM NaCl, 1.5 mM MgCl2, 1 mM EDTA, 10% glycerol, 1% Triton X-100) containing protease inhibitors. The lysates were centrifuged at 15,000 ×g for 10 min at 4 °C, and the supernatant used. The protein concentration of the supernatant was measured by the Coomassie Plus protein quantification method (Thermo Fisher Scientific, USA). For western blotting analysis, equal amounts of protein (50 µg) of each sample were boiled in reducing Laemmli sample buffer and resolved by 8-10% SDS-PAGE. Proteins were then transferred onto Immobilon-P membranes (Millipore Corporation, MA, USA) blocked with 5% non-fat milk in TBST buffer, and incubated with selected primary antibody: acetylated-FoxO1, forkhead box protein O1 (FoxO1), SIRT1, proliferator-activated receptor gamma, coactivator 1 alpha (PGC1α) (Santa Cruz, TX, USA), NF-κB, phosphorylated NF-κB and CYP2E1 (Cell signaling, MA, USA), and respective second antibodies (Santa Cruz, TX, USA). Proteins were detected by a horseradish peroxidase-conjugated secondary antibody (Bio-Rad, CA, USA). The specific bands were visualized by a Super Signal West Pico Chemiluminescent Substrate Kit (Pierce, IL, USA) according to the manufacturer’s instructions. glyceraldehyde-3-phosphate dehydrogenase (GAPDH) antibody was used as internal control. Intensities of protein bands were quantified using GS-710 Calibrated Imaging Densitometer (Bio-Rad, CA, USA).
RNA extraction and quantitative real-time PCR (qRT-PCR)
Total RNA was extracted from frozen liver sections with Trizol reagent (Invitrogen, NY, USA), as previously described (15). cDNA was prepared from the RNA samples using M-MLV reverse transcriptase (Invitrogen, NY, USA) and an automated thermal cycler PTC-200 (MJ Research, USA). qRT-PCR was performed using FastStart Universal SYBR Green Master (ROX) (Roche, USA). Relative gene expression was determined using the 2-∆∆CT method. The sequences for TNFα, IL-6 and IL-1β were: tumor necrosis factor alpha (Tnf-α) forward-CAAACCACCAAGTGGAGGAG, reverse-CGGACTCCGCAAAGTCTAAG; IL-6 forward-GGATACCACTCCCAACAGACCT, reverse-GCCATTGCACAACTCTTTTCTC; IL-1β forward-AGCCAAGCTTCCTTGTGCAAGTGT, reverse-GCAGCCCTTCATCTTTTGGGGTCC. GAPDH was used as internal control, and the sequences were: forward 5'-ATCACCATCTTCCAGGAGCGA-3' and reverse 5'-AGCCTTCTCCATGGTGGTGAA-3'.
Hepatic NAD/NADH ratio evaluation
Quantification of oxidized nicotinamide adenine dinucleotide (NAD) and reduced nicotinamide adenine dinucleotide (NADH) in the liver was carried out using NAD/NADH assay kit from BioVision (Milpitas, CA, USA), according to the manufacturer’s instructions. Briefly, livers were snap frozen in liquid nitrogen and extracted at 4 °C with extraction buffer provided with the NAD/NADH assay kit. NAD and NADH values were normalized by protein concentration.
Statistical analysis
Graph Pad Prism (Graph Pad Software, CA, USA) was used to perform the statistical analysis, and resulting values were expressed as mean ± SEM or mean ± SD, as indicated in the footnotes. Group means were compared using one-way ANOVA analysis with adjustments for multiple comparisons. P value was set at 0.05 for the comparisons to reach statistical significance. Different letters indicate significant differences between groups.
Results
Luteolin inhibited steatosis and DEN-initiated ethanol promoted hepatic carcinogenesis
Ethanol fed groups had significantly lower final body weight and significantly higher liver weight in comparison to Control and Control + DEN groups (Table 1). Luteolin had no significant effect on final body weight or liver weight when comparing DEN + EtOH and DEN + EtOH + L groups (Table 1).
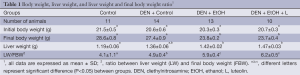
Full table
Regarding pathology data, all mice in DEN + EtOH group developed steatosis (62% in grade 1 and 39% in grade 2, Figure 2). Luteolin inhibited steatosis in DEN + EtOH + L group, in which 20% of mice presented steatosis in grade 2, 70% with grade 1 and 10% had no steatosis (Figure 2). In DEN injection group without ethanol feeding, one animal presented steatosis in grade 1 (DEN + Control, Figure 2) and steatosis was not detected in the Control group. Similarly, preneoplastic foci, markers of precancerous lesions, were not observed in the absence of ethanol in DEN-injection group (DEN + Control, Figure 3A). DEN + EtOH group showed incidence of 54% of preneoplastic foci (Figure 3A). Preneoplastic foci were not detected after luteolin supplementation (DEN + EtOH + L group and in Control and DEN + Control groups (Figure 3A).
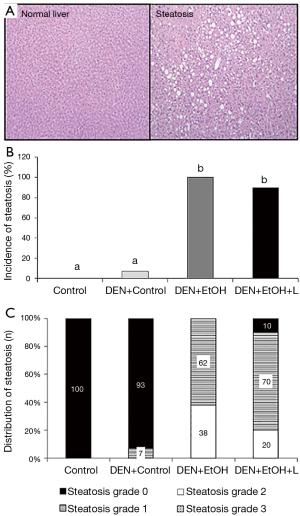

Luteolin reduces hepatic inflammation in DEN-initiated EtOH-promoted hepatic carcinogenesis
DEN + EtOH group had a significantly greater number of inflammatory foci as compared to Control and DEN + Control groups (by approximately 5 fold, Figure 3B). Luteolin supplementation significantly reduced by 50% the number of hepatic inflammatory foci in comparison to DEN + EtOH group (Figure 3B). Regarding inflammatory cytokines, no changes in Tnf-α and Il-6 mRNA expression were detected between all groups. mRNA expression of Il-1β was significantly higher in DEN + Control group in comparison to Control group. DEN + EtOH + L group presented significantly lower Il-1β mRNA expression as compared to DEN + Control group, as shown in Figure 4. Western blot analysis was conducted to evaluate total NF-κB protein expression, but no differences were observed among the groups (data not shown).
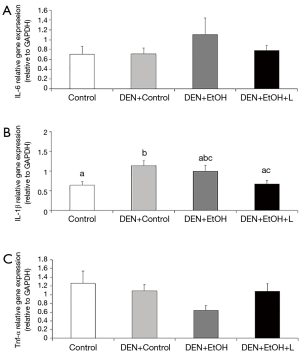
Reduction in DEN-initiated EtOH-promoted hepatic carcinogenesis by luteolin was associated with restored SIRT1 activity and increased PGC1α protein expression
Western blot analyses were conducted to evaluate SIRT1 protein expression and activity in the liver, as well as its downstream target PGC1α. To evaluate SIRT1 activity, the acetylation of FoxO1 was determined. No difference in SIRT1 protein expression was observed among the groups (Figure 5A). DEN-EtOH group showed decreased SIRT1 deacetylase activity (Figure 5B) in comparison to Control group, as represented by the increased acetylated FoxO1 ratio, and diminished PGC1α protein expression (Figure 5C). Luteolin supplementation significantly increased SIRT1 deacetylase activity and PGC1α protein expression in DEN + EtOH + L group as compared to DEN + EtOH group (Figure 5B,C). No changes in NAD+/NADH ratio in the liver were observed among all groups (data not shown).
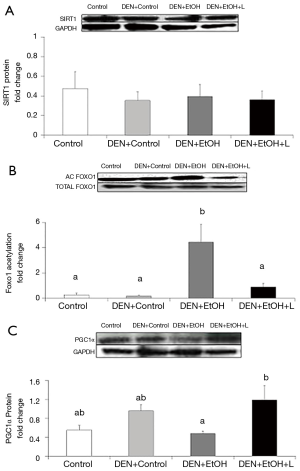
CYP2E1 protein expression was significantly increased in the liver of both ethanol fed groups (DEN + EtOH and DEN + EtOH + L) in comparison with Control and DEN + Control groups, and luteolin did not affect its expression (data not shown).
Discussion
In the present study, ethanol treatment caused a significant increase in preneoplastic lesions associated with steatosis and inflammatory foci after 9 weeks of exposure in DEN-initiated mice. The absence of preneoplastic lesions in the Control mice given the same dose of the DEN carcinogen alone suggests the promoting effect of alcohol intake on hepatic carcinogenesis in this model. This notion was supported by our previous study in a rat model (35). Furthermore, the significantly decreased final body weight and significantly increased liver weight found in DEN initiated ethanol fed groups provided evidence of the deleterious effect of alcohol intake in growth and liver function in this model.
To our knowledge this is the first report showing that treatment with luteolin significantly inhibited DEN-initiated alcohol-promoted liver pre-cancerous lesions associated with inhibition of steatosis and inflammatory foci in mice. Besides the promotion of carcinogenesis, the effects of long-term alcohol intake include a number of biochemical and molecular alterations and pathological lesions such as steatosis (4,35). The anti-steatosis effect of luteolin reported in the present study is supported by previous reports showing that luteolin and herb extracts containing luteolin can inhibit lipid accumulation in the liver (46,47). Our findings also complement previous studies with cell lines and different murine models showing that luteolin has anti-cancer (29,32,48-50) and anti-inflammatory properties (29,51,52). It is also important to note that the protective effect of luteolin was not associated with changes in the body weight of mice, suggesting minimal toxicity of luteolin intake.
It is well known that carcinogenesis is a process that involves the transition of a normal cell into a preneoplastic lesion that might develop into a malignant tumor, but the precise mechanism of alcohol-associated carcinogenesis is not fully understood. In our findings, the presence of preneoplastic lesions in DEN + EtOH group was associated with a significant increase in the number of inflammatory foci and IL-1β, suggesting the role of inflammation in the progression of cancer in this model (11). To support this concept, hepatic inflammatory foci have been shown to have a good correlation with carcinogenicity (45,53). Luteolin treatment inhibited inflammatory foci and reduced gene expression of IL-1β and IL-6 in comparison to DEN + EtOH group. TNF-α expression was not increased in DEN + EtOH group, but it is important to note that luteolin restored its gene expression to levels similar to the control group. Therefore, the anti-inflammatory properties of luteolin could be related to the anti-carcinogenic effect observed in this work. This notion is supported by Lin et al. (28) that postulated that the anti-carcinogenic potential of luteolin is related to reduced TNFα and IL-1 by inhibition of NF-κB signaling. In this study, we did not detect changes in NF-κB protein expression and the effect found in inflammatory cytokine expression, such as TNFα was marginal. This could be due to the use of homogenates of hepatic tissue with varying sites of lesions that might make the differences difficult to detect.
In order to explore the mechanisms by which luteolin prevents alcohol induced liver injury, we evaluated the SIRT1protein expression and deacetylase activity. In the present study, DEN-EtOH group presented an increase in FoxO1 acetylation and a decrease in SIRT 1 downstream target PGC1α protein expression, suggesting decreased SIRT1 deacetylase activity. This finding is supported by a previous work of our laboratory showing that alcohol intake impairs the function of SIRT1 (16). Interestingly, the protective effect of luteolin was accompanied by induction of SIRT1 activity, demonstrated by decreased acetylation of FoxO1 and increased PGC1α protein expression.
Decreased SIRT1 activity has been linked to chronic inflammation, and it was previously reported in liver cancer (54). SIRT1 regulates many cellular processes through deacetylating FoxO family transcriptor factors, such as FoxO1 (55). Decreased SIRT1 deacetylation leads to increased FoxO1 expression, enhancing inflammation by affecting the transcription of pro-inflammatory genes like TNFα and IL-6 (54). Our results are in accordance with these observations, since DEN + EtOH group showed increased acetylated FoxO1 in association with inflammatory response. Luteolin seems to inhibit inflammation through SIRT1 activation, since the FoxO1 acetylation and inflammatory foci were decreased in luteolin treated mice. No previous studies have been found about luteolin and sirtuins, but similar results are described for resveratrol, a flavonoid considered a SIRT1 activator, with anti-inflammatory and anti-carcinogenic action (56-59).
PGC-1α is the master regulator of mitochondrial biogenesis and function (60,61). SIRT1 deacetylates PGC-1a, enhancing PGC1α activity (60,62). In the present study, decreased SIRT1 activity observed in the DEN + EtOH group was accompanied by decreased PGC1α protein expression. This observation is supported by previous reports showing that overexpression of SIRT1 in livers of mice significantly increases the expression of genes that are under the control of PGC-1α, whereas SIRT1 knockdown attenuated this effect (63). Deletion of SIRT1 can alter fatty acid metabolism in the liver and PGC1α can activate the oxidative metabolism by increasing mitochondrial biogenesis and increasing fatty acid oxidation (61,63-65). In the present study, the diminished SIRT1-PGC1α signaling could be associated to the increased steatosis found after ethanol feeding in DEN initiated mice. Decreased PGC1α expression has also been reported in a variety of cancers, and PGC1α seems to regulate a crosstalk between energy metabolism and inflammation (66). Some reports suggest that overexpression of PGC1α can suppress the secretion of pro inflammatory cytokines (65,67). The increased SIRT1 activity induced by luteolin could activate PGC1α, reduce steatosis and inflammation caused by DEN and ethanol, thus contributing to the anti-tumorigenic action found in this study. Similarly, Wang and colleagues described that activation of SIRT1 by resveratrol treatment reduced tumorigenesis in mice, associated with increased levels of PGC1α (68).
In order to investigate how luteolin can affect SIRT1 activity, we evaluated NAD+/NADH ratio in the liver. SIRT1 function is tightly regulated by NAD+ availability (54), and this availability can be reduced by alcohol oxidation (3,69). However, the decreased SIRT1 activity found in our study seems to be mediated by a different mechanism, since no differences on NAD+/NADH ratio was observed between all groups.
Since CYP2E1 is an important pathway of ethanol metabolism, and its induction by chronic alcohol intake results in an enhanced activation of procarcinogens to carcinogens and release of inflammatory mediators (70), we evaluated the role of CYP2E1 expression in the present study. DEN initiated ethanol fed mice showed significantly increased protein expression of the enzyme as compared to control diet groups, but luteolin did not reverse CYP2E1 expression. These data support our notion that the potential protective effect of luteolin is related to SIRT1 signaling.
Conclusions
This study demonstrated that luteolin prevents alcohol promoted hepatic carcinogenesis potentially mediated by a SIRT1 signaling pathway. Our findings support the potential use of dietary means for preventing alcohol induced liver lesions and open a new avenue for research on interaction between luteolin and SIRT1.
Acknowledgements
The authors would like to thank Dr. Donald Smith for his assistance in animal study and Ms. Junrui Cheng for her assistance on this manuscript.
Funding: This study was supported by US Department of Agriculture grant 1950-51000-074S and the NIH/NCI CA176256 grant. Any opinions, findings, conclusions, and recommendations expressed in this publication are those of the author(s) and do not necessarily reflect the views of the sponsors. Bruna Paola Murino Rafacho received Research Internships Abroad (BEPE) fellowship from Sao Paulo Research Foundation (FAPESP, Process number: FAPESP 2012/23759-0).
Disclosure: The authors declare no conflict of interest.
Ethical Statement: All animal protocols were approved by the Institutional Animal Care and Use Committee at the Jean Mayer-USDA Human Nutrition Research Center on Aging at Tufts University.
References
- Tian H, Ge C, Li H, et al. Ribonucleotide reductase M2B inhibits cell migration and spreading by early growth response protein 1-mediated phosphatase and tensin homolog/Akt1 pathway in hepatocellular carcinoma. Hepatology 2014;59:1459-70. [PubMed]
- Ye Q, Lian F, Chavez PR, et al. Cytochrome P450 2E1 inhibition prevents hepatic carcinogenesis induced by diethylnitrosamine in alcohol-fed rats. Hepatobiliary Surg Nutr 2012;1:5-18. [PubMed]
- Zakhari S. Overview: how is alcohol metabolized by the body? Alcohol Res Health 2006;29:245-54. [PubMed]
- Orman ES, Odena G, Bataller R. Alcoholic liver disease: pathogenesis, management, and novel targets for therapy. J Gastroenterol Hepatol 2013;28 Suppl 1:77-84. [PubMed]
- Takada A, Matsuda Y, Takase S. Effects of dietary fat on alcohol-pyrazole hepatitis in rats: the pathogenetic role of the nonalcohol dehydrogenase pathway in alcohol-induced hepatic cell injury. Alcohol Clin Exp Res 1986;10:403-11. [PubMed]
- Brandon-Warner E, Walling TL, Schrum LW, et al. Chronic ethanol feeding accelerates hepatocellular carcinoma progression in a sex-dependent manner in a mouse model of hepatocarcinogenesis. Alcohol Clin Exp Res 2012;36:641-53. [PubMed]
- Seitz HK, Stickel F. Molecular mechanisms of alcohol-mediated carcinogenesis. Nat Rev Cancer 2007;7:599-612. [PubMed]
- Homann N, Seitz HK, Wang XD, et al. Mechanisms in alcohol-associated carcinogenesis. Alcohol Clin Exp Res 2005;29:1317-20. [PubMed]
- Kew MC. The role of cirrhosis in the etiology of hepatocellular carcinoma. J Gastrointest Cancer 2014;45:12-21. [PubMed]
- Purohit V, Rapaka R, Kwon OS, et al. Roles of alcohol and tobacco exposure in the development of hepatocellular carcinoma. Life Sci 2013;92:3-9. [PubMed]
- Aravalli RN. Role of innate immunity in the development of hepatocellular carcinoma. World J Gastroenterol 2013;19:7500-14. [PubMed]
- Drexler SK, Yazdi AS. Complex roles of inflammasomes in carcinogenesis. Cancer J 2013;19:468-72. [PubMed]
- Seitz HK, Wang XD. The role of cytochrome P450 2E1 in ethanol-mediated carcinogenesis. Subcell Biochem 2013;67:131-43. [PubMed]
- Braicu C, Burz C, Berindan-Neagoe I, et al. Hepatocellular Carcinoma: Tumorigenesis and Prediction Markers. Gastroenterology Res 2009;2:191-9.
- Ip BC, Hu KQ, Liu C, et al. Lycopene metabolite, apo-10'-lycopenoic acid, inhibits diethylnitrosamine-initiated, high fat diet-promoted hepatic inflammation and tumorigenesis in mice. Cancer Prev Res (Phila) 2013;6:1304-16. [PubMed]
- Nascimento AF, Ip BC, Luvizotto RA, et al. Aggravation of nonalcoholic steatohepatitis by moderate alcohol consumption is associated with decreased SIRT1 activity in rats. Hepatobiliary Surg Nutr 2013;2:252-9. [PubMed]
- Wang Y, Seitz HK, Wang XD. Moderate alcohol consumption aggravates high-fat diet induced steatohepatitis in rats. Alcohol Clin Exp Res 2010;34:567-73. [PubMed]
- Kume S, Haneda M, Kanasaki K, et al. Silent information regulator 2 (SIRT1) attenuates oxidative stress-induced mesangial cell apoptosis via p53 deacetylation. Free Radic Biol Med 2006;40:2175-82. [PubMed]
- Li Y, Xu S, Giles A, et al. Hepatic overexpression of SIRT1 in mice attenuates endoplasmic reticulum stress and insulin resistance in the liver. FASEB J 2011;25:1664-79. [PubMed]
- Zhang C, Feng Y, Qu S, et al. Resveratrol attenuates doxorubicin-induced cardiomyocyte apoptosis in mice through SIRT1-mediated deacetylation of p53. Cardiovasc Res 2011;90:538-45. [PubMed]
- Herranz AS, Gonzalo-Gobernado R, Reimers D, et al. Applications of human umbilical cord blood cells in central nervous system regeneration. Curr Stem Cell Res Ther 2010;5:17-22. [PubMed]
- Portmann S, Fahrner R, Lechleiter A, et al. Antitumor effect of SIRT1 inhibition in human HCC tumor models in vitro and in vivo. Mol Cancer Ther 2013;12:499-508. [PubMed]
- Yuan H, Su L, Chen WY. The emerging and diverse roles of sirtuins in cancer: a clinical perspective. Onco Targets Ther 2013;6:1399-416. [PubMed]
- Chen HC, Jeng YM, Yuan RH, et al. SIRT1 promotes tumorigenesis and resistance to chemotherapy in hepatocellular carcinoma and its expression predicts poor prognosis. Ann Surg Oncol 2012;19:2011-9. [PubMed]
- López-Lázaro M. Distribution and biological activities of the flavonoid luteolin. Mini Rev Med Chem 2009;9:31-59. [PubMed]
- Kushi LH, Doyle C, McCullough M, et al. American Cancer Society Guidelines on nutrition and physical activity for cancer prevention: reducing the risk of cancer with healthy food choices and physical activity. CA Cancer J Clin 2012;62:30-67. [PubMed]
- Wang L, Lee IM, Zhang SM, et al. Dietary intake of selected flavonols, flavones, and flavonoid-rich foods and risk of cancer in middle-aged and older women. Am J Clin Nutr 2009;89:905-12. [PubMed]
- Lin Y, Shi R, Wang X, et al. Luteolin, a flavonoid with potential for cancer prevention and therapy. Curr Cancer Drug Targets 2008;8:634-46. [PubMed]
- Domitrović R, Cvijanović O, Pugel EP, et al. Luteolin ameliorates cisplatin-induced nephrotoxicity in mice through inhibition of platinum accumulation, inflammation and apoptosis in the kidney. Toxicology 2013;310:115-23. [PubMed]
- Ju W, Wang X, Shi H, et al. A critical role of luteolin-induced reactive oxygen species in blockage of tumor necrosis factor-activated nuclear factor-kappaB pathway and sensitization of apoptosis in lung cancer cells. Mol Pharmacol 2007;71:1381-8. [PubMed]
- Kapoor S. Luteolin and its inhibitory effect on tumor growth in systemic malignancies. Exp Cell Res 2013;319:777-8. [PubMed]
- Balamurugan K, Karthikeyan J. Evaluation of Luteolin in the Prevention of N-nitrosodiethylamine-induced Hepatocellular Carcinoma Using Animal Model System. Indian J Clin Biochem 2012;27:157-63. [PubMed]
- Anderson LM, Carter JP, Driver CL, et al. Enhancement of tumorigenesis by N-nitrosodiethylamine, N-nitrosopyrrolidine and N6-(methylnitroso)-adenosine by ethanol. Cancer Lett 1993;68:61-6. [PubMed]
- Anderson LM, Carter JP, Logsdon DL, et al. Characterization of ethanol’s enhancement of tumorigenesis by N-nitrosodimethylamine in mice. Carcinogenesis 1992;13:2107-11. [PubMed]
- Chavez PR, Lian F, Chung J, et al. Long-term ethanol consumption promotes hepatic tumorigenesis but impairs normal hepatocyte proliferation in rats. J Nutr 2011;141:1049-55. [PubMed]
- Samy RP, Gopalakrishnakone P, Ignacimuthu S. Anti-tumor promoting potential of luteolin against 7,12-dimethylbenz(a)anthracene-induced mammary tumors in rats. Chem Biol Interact 2006;164:1-14. [PubMed]
- Seelinger G, Merfort I, Wölfle U, et al. Anti-carcinogenic effects of the flavonoid luteolin. Molecules 2008;13:2628-51. [PubMed]
- Shimoi K, Okada H, Furugori M, et al. Intestinal absorption of luteolin and luteolin 7-O-beta-glucoside in rats and humans. FEBS Lett 1998;438:220-4. [PubMed]
- Li LP, Wu XD, Chen ZJ, et al. Interspecies difference of luteolin and apigenin after oral administration of Chrysanthemum morifolium extract and prediction of human pharmacokinetics. Pharmazie 2013;68:195-200. [PubMed]
- Gates MA, Tworoger SS, Hecht JL, et al. A prospective study of dietary flavonoid intake and incidence of epithelial ovarian cancer. Int J Cancer 2007;121:2225-32. [PubMed]
- Sampson L, Rimm E, Hollman PC, et al. Flavonol and flavone intakes in US health professionals. J Am Diet Assoc 2002;102:1414-20. [PubMed]
- Sharma V, McNeill JH. To scale or not to scale: the principles of dose extrapolation. Br J Pharmacol 2009;157:907-21. [PubMed]
- Reagan-Shaw S, Nihal M, Ahmad N. Dose translation from animal to human studies revisited. FASEB J 2008;22:659-61. [PubMed]
- Chung J, Koo K, Lian F, et al. Apo-10'-lycopenoic acid, a lycopene metabolite, increases sirtuin 1 mRNA and protein levels and decreases hepatic fat accumulation in ob/ob mice. J Nutr 2012;142:405-10. [PubMed]
- Bannasch P, Haertel T, Su Q. Significance of hepatic preneoplasia in risk identification and early detection of neoplasia. Toxicol Pathol 2003;31:134-9. [PubMed]
- Liu C, Bronson RT, Russell RM, et al. β-Cryptoxanthin supplementation prevents cigarette smoke-induced lung inflammation, oxidative damage, and squamous metaplasia in ferrets. Cancer Prev Res (Phila) 2011;4:1255-66. [PubMed]
- Davaatseren M, Hur HJ, Yang HJ, et al. Taraxacum official (dandelion) leaf extract alleviates high-fat diet-induced nonalcoholic fatty liver. Food Chem Toxicol 2013;58:30-6. [PubMed]
- Amin AR, Wang D, Zhang H, et al. Enhanced anti-tumor activity by the combination of the natural compounds (-)-epigallocatechin-3-gallate and luteolin: potential role of p53. J Biol Chem 2010;285:34557-65. [PubMed]
- Lee EJ, Oh SY, Sung MK. Luteolin exerts anti-tumor activity through the suppression of epidermal growth factor receptor-mediated pathway in MDA-MB-231 ER-negative breast cancer cells. Food Chem Toxicol 2012;50:4136-43. [PubMed]
- Hwang JT, Park OJ, Lee YK, et al. Anti-tumor effect of luteolin is accompanied by AMP-activated protein kinase and nuclear factor-κB modulation in HepG2 hepatocarcinoma cells. Int J Mol Med 2011;28:25-31. [PubMed]
- Kim JE, Son JE, Jang YJ, et al. Luteolin, a novel natural inhibitor of tumor progression locus 2 serine/threonine kinase, inhibits tumor necrosis factor-alpha-induced cyclooxygenase-2 expression in JB6 mouse epidermis cells. J Pharmacol Exp Ther 2011;338:1013-22. [PubMed]
- Seelinger G, Merfort I, Schempp CM. Anti-oxidant, anti-inflammatory and anti-allergic activities of luteolin. Planta Med 2008;74:1667-77. [PubMed]
- Kochi T, Shimizu M, Terakura D, et al. Non-alcoholic steatohepatitis and preneoplastic lesions develop in the liver of obese and hypertensive rats: suppressing effects of EGCG on the development of liver lesions. Cancer Lett 2014;342:60-9. [PubMed]
- Liu TF, McCall CE. Deacetylation by SIRT1 Reprograms Inflammation and Cancer. Genes Cancer 2013;4:135-47. [PubMed]
- Olmos Y, Brosens JJ, Lam EW. Interplay between SIRT proteins and tumour suppressor transcription factors in chemotherapeutic resistance of cancer. Drug Resist Updat 2011;14:35-44. [PubMed]
- Lin HC, Chen YF, Hsu WH, et al. Resveratrol helps recovery from fatty liver and protects against hepatocellular carcinoma induced by hepatitis B virus X protein in a mouse model. Cancer Prev Res (Phila) 2012;5:952-62. [PubMed]
- de la Lastra CA, Villegas I. Resveratrol as an anti-inflammatory and anti-aging agent: mechanisms and clinical implications. Mol Nutr Food Res 2005;49:405-30. [PubMed]
- Singh NP, Singh UP, Hegde VL, et al. Resveratrol (trans-3,5,4'-trihydroxystilbene) suppresses EL4 tumor growth by induction of apoptosis involving reciprocal regulation of SIRT1 and NF-κB. Mol Nutr Food Res 2011;55:1207-18. [PubMed]
- Villalba JM, Alcaín FJ. Sirtuin activators and inhibitors. Biofactors 2012;38:349-59. [PubMed]
- Jeninga EH, Schoonjans K, Auwerx J. Reversible acetylation of PGC-1: connecting energy sensors and effectors to guarantee metabolic flexibility. Oncogene 2010;29:4617-24. [PubMed]
- Austin S, St-Pierre J. PGC1α and mitochondrial metabolism--emerging concepts and relevance in ageing and neurodegenerative disorders. J Cell Sci 2012;125:4963-71. [PubMed]
- Preyat N, Leo O. Sirtuin deacylases: a molecular link between metabolism and immunity. J Leukoc Biol 2013;93:669-80. [PubMed]
- Rodgers JT, Puigserver P. Fasting-dependent glucose and lipid metabolic response through hepatic sirtuin 1. Proc Natl Acad Sci U S A 2007;104:12861-6. [PubMed]
- Sugden MC, Caton PW, Holness MJ. PPAR control: it's SIRTainly as easy as PGC. J Endocrinol 2010;204:93-104. [PubMed]
- Purushotham A, Schug TT, Xu Q, et al. Hepatocyte-specific deletion of SIRT1 alters fatty acid metabolism and results in hepatic steatosis and inflammation. Cell Metab 2009;9:327-38. [PubMed]
- Kauppinen A, Suuronen T, Ojala J, et al. Antagonistic crosstalk between NF-κB and SIRT1 in the regulation of inflammation and metabolic disorders. Cell Signal 2013;25:1939-48. [PubMed]
- Kim HJ, Park KG, Yoo EK, et al. Effects of PGC-1alpha on TNF-alpha-induced MCP-1 and VCAM-1 expression and NF-kappaB activation in human aortic smooth muscle and endothelial cells. Antioxid Redox Signal 2007;9:301-7. [PubMed]
- Wang RH, Sengupta K, Li C, et al. Impaired DNA damage response, genome instability, and tumorigenesis in SIRT1 mutant mice. Cancer Cell 2008;14:312-23. [PubMed]
- Pöschl G, Stickel F, Wang XD, et al. Alcohol and cancer: genetic and nutritional aspects. Proc Nutr Soc 2004;63:65-71. [PubMed]
- Mueller S. Pharmacological blockage of CYP2E1 and alcohol-mediated liver cancer: is the time ready? Chin J Cancer Res 2013;25:269-71. [PubMed]