Sirtuin 1 signaling and alcoholic fatty liver disease
Role of sirtuin 1 (SIRT1) in alcoholic fatty liver disease (AFLD)
SIRT1 is a nicotinamide adenine dinucleotide (NAD+, NADH)-dependent class III histone deacetylase and acts as part of the deacetylation reaction, producing nicotinamide (NAM, vitamin B3) and acetyl-ADP-ribose (1-5). Liver is one of the key organs where SIRT1 plays a pivotal role in the regulation of lipid metabolism and inflammation by modifying the acetylation status of various target molecules, including histones, transcriptional regulators, and its co-regulators (1-5).
In human, chronic or acute alcohol intoxication is commonly associated with several forms of liver injuries, including excess accumulation of fat within the liver (steatosis), inflammation (hepatitis), fibrosis/cirrhosis, cancer, and can eventually cause liver failure (6). Considerable evidences from rodent and human studies demonstrate that disruption of the hepatic SIRT1 signaling by ethanol plays a central role in the development of AFLD (7-21). Ethanol down-regulates SIRT1 in hepatic cells and in animal liver. The ethanol-mediated disruption of SIRT1 signaling leads to excess fat accumulation and inflammatory responses in the liver of animals and humans. Treatment with resveratrol, a known SIRT1 agonist, can alleviate liver steatosis and normalize elevated levels of serum alanine aminotransferase (ALT), which is a marker of liver injury, in chronically ethanol-fed mice (10,22). Stimulating SIRT1 activity by SIRT1wt overexpression in hepatocytes prevents fat accumulation in a cellular model of alcoholic steatosis (20). Genetic specific ablation of liver SIRT1 [liver specific knockout (Sirt1LKO) mice] is associated with rapid onset and progression of steatosis and inflammation in response to chronic-binge ethanol challenge (20). Strikingly, ethanol-administrated Sirt1LKO mice partially progressed from fatty liver to mild fibrotic liver (20). These findings suggest an essential role of SIRT1 signaling in AFLD.
Nutritional or pharmacological modification of SIRT1 prevents AFLD in animals
Growing evidence has demonstrated that stimulation of hepatic SIRT1 signaling by nutritional or pharmacological intervention protects against the development of AFLD in rodents. Treatment with resveratrol, a dietary polyphenol found in grapes, red wines and berries, stimulated SIRT1 signaling and alleviated alcoholic liver steatosis in mice (10). Consumption of high dietary content of saturated fatty acids, via up-regulation of SIRT1, protected against the development of liver steatosis in mice fed with ethanol (9,14). Supplementation of ethanol-containing diets with a peroxisome proliferator-activated receptor γ (PPARγ) agonist, rosiglitazone, attenuated hepatic lipid accumulation in mice by turning on SIRT1 signaling via inducing adiponectin, which is one of the adipocyte-derived hormones (13). Supplement of salvianolic acid B, an antioxidant isolated from the traditional Chinese medical herb Salvia miltiorrhiza, ameliorated acute alcohol-induced liver injury through modulating SIRT1-p53 axis in rats (21). These findings suggest that novel strategies to stimulate hepatic SIRT1 activity may serve as potential therapies for treating human AFLD.
Ethanol disrupts hepatic SIRT1 signaling
The molecular mechanisms of ethanol-mediated disruption of SIRT1 signaling and subsequent development of liver steatosis, inflammation, and mild fibrosis are still not fully understood. However, accumulating evidence demonstrates that ethanol-mediated SIRT1 inhibition leads to development of AFLD largely through disruption of a signaling network mediated by various transcriptional regulators and co-regulators, including AMP-activated kinase (AMPK), sterol regulatory element-binding protein 1 (SREBP-1), peroxisome proliferator-activated receptor α (PPARα), PPARγ co-activator-1α (PGC-1α), lipin-1, forkhead transcription factor O1 (FoxO1), β-catenin, nuclear transcription factor-κB (NF-κB), and nuclear factor activated T cells c4 (NFATc4) (Figure 1). Disruption of this signaling network by ethanol via SIRT1 inhibition ultimately leads to steatosis and over production of inflammatory cytokines in liver (7-21).
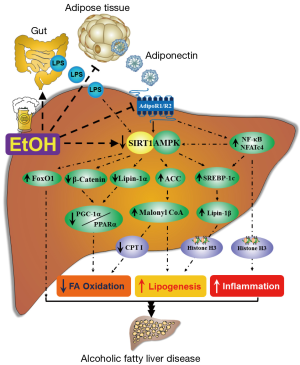
SIRT1-AMPK signaling system and AFLD
Ethanol-mediated dysregulation of hepatic AMPK, a vital lipid regulator, is one of the major mechanisms for AFLD (10,13,19,23-31). Hepatic AMPK inhibition induced by ethanol exposure leads to stimulated acetyl-CoA carboxylase (ACC), resulting in an induction of malonyl-CoA, which is a precursor for biosynthesis of fatty acids, and suppression of carnitine palmitoytransferase I (CPT-I), the enzyme that controls the transfer of long-chain fatty acyl-CoA into mitochondria. Ethanol-mediated impairment of AMPK signaling promotes lipid accumulation and halts lipid catabolism, which ultimately causes the development of AFLD in animals.
Accumulating evidences suggest that SIRT1 and AMPK regulate each other, share similar signaling pathways, and modulate many common targets (32). Studies in cultured hepatic cells and in animal liver have provided evidence that SIRT1 is able to stimulate AMPK activity via modulation of liver kinase B1 (LKB1), an upstream AMPK kinase (33,34). On the other hand, activation of AMPK via LKB-1 leads to increased cellular NAD+ levels, which subsequently activates SIRT1 signaling (35,36). Thus, this unique SIRT1-AMPK axis participates in regulating various lipid metabolism and inflammation pathways (32-36).
Resveratrol, a known agonist of both SIRT1 and AMPK, exerts its protective action against AFLD by turning on the hepatic SIRT1-AMPK axis in mice (10). An adipocyte-derived hormone, adiponectin, alleviates steatohepatitis largely by stimulating hepatic SIRT1-AMPK axis in both cellular and animal models of AFLD (13,25). Thus, deregulation of SIRT1-AMPK axis by ethanol represents an attractive hypothesis of impaired central upstream signaling system for AFLD.
SIRT1-SREBP-1 axis and AFLD
Activation of SREBP-1 by ethanol is pivotal to pathogenesis of AFLD. Increase in hepatic SREBP-1c activity has been found in various alcoholic steatosis models including cultured hepatocytes, zebrafish, mice or micropig (9,10,25,27,37-44). Both acute and chronic ethanol exposure stimulated SREBP-1 activity and increased the gene and protein expression of a panel of SREBP-1-regulated enzymes including fatty acid synthase (FAS), stearoyl-CoA desaturase (SCD), mitochondrial glycerol-3-phosphate acyltransferase 1 (GPAT1), malic enzyme (ME), ATP citrate lyase (ACL), and ACC, and led to hepatic excessive lipid accumulation. More importantly, SREBP-1 knockout mice were completely protected from AFLD, indicating a causal involvement of SREBP-1 in AFLD (41). On the contrary, the development of alcoholic liver steatosis was also observed in the model of suppressed SREBP-1c signaling in rats, implying that the effects of ethanol on SREBP-1 might vary with species (45,46).
Several line of evidence demonstrates the interplay between SIRT1 and SREBP-1 (7,47,48). SREBP-1c protein stability and activity were dynamically regulated by reversible acetylation via histone acetyltransferase (HAT), CBP/p300, and SIRT1. SIRT1 regulates SREBP-1c activity by deacetylating SREBP-1c and by inhibiting its transcriptional activity, resulting in transcriptional repression of the SREBP-1c-targeted lipogenic enzymes such as FAS, SCD, GPAT1, ME, ACL, and ACC in cultured hepatocytes and mouse liver (7,47,48). The acetylation level of SREBP-1 was highly elevated in hepatic cells exposed to ethanol or ethanol-fed mice. Inhibition of SIRT1 activity by ethanol feeding was associated with an increase in the acetylated active nuclear form of SREBP-1c, and consequently leads to the development of steatosis (7). More importantly, overexpression of SIRT1wt or treatment with resveratrol alleviated AFLD by reducing the ethanol-induced increases in SREBP-1c hyperacetylation levels and SREBP-1 activity (7,10). Therefore, the ability of ethanol to modulate SIRT1-SREBP-1c axis has been proposed as one of the underlying mechanisms linking ethanol exposure with hepatic lipogenic gene expression and AFLD development. Interestingly, ethanol-mediated SREBP-1 activation is partially mediated via AMPK inhibition, suggesting the regulation of SREBP-1 activity by SIRT1 either via AMPK dependent- or independent-mechanisms in the development of AFLD (23).
SIRT1-PGC-1α/PPARα axis and AFLD
PGC-1α is a prominent transcriptional regulator of lipid metabolism. SIRT1 directly interacts with, and deacetylates PGC-1α, which subsequently modulates PGC-1α activity (49-51). SIRT1 has been identified as a functional regulator of PGC-1α that activates a metabolic gene transcription program of mitochondrial fatty acid oxidation. Interestingly, the acetylation status of PGC-1α is generally considered as an in vivo marker of SIRT1 activity (50,51).
The role of PGC-1α in the development of AFLD in rodents has been unequivocally established (7,9,10,13,14,19,20,52,53). Severely reduced hepatic PGC-1α gene and protein expression occurred consistently in ethanol-fed mice. Moreover, ethanol administration to mice significantly increased the ratio of acetylated PGC-1α to total PGC-1α protein in mouse livers (7,9,10). We have recently found that removal of hepatic lipin-1 from mice augmented the ethanol-induced impairment of hepatic fatty acid oxidation and lipoprotein production, largely by deactivating hepatic PGC-1α (53).
PGC-1α co-activates with PPARα to induce expression of mitochondrial fatty acid oxidation enzymes (50). SIRT1 regulates lipid homeostasis by positively regulating PPARα (1,50). Hepatocyte-specific deletion of SIRT1 disturbs PPARα signaling, reduces fatty acid oxidation, and causes aggravated liver steatosis and inflammation. Impairments of both PGC-1α and PPARα have been implicated in the development of AFLD in animals (13,19,45,52-61). Therefore, it is likely that disruption of SIRT1-PGC-1α/PPARα axis by ethanol may act as one of the main triggers of AFLD.
SIRT1-lipin-1 axis and AFLD
Lipin-1, a mammalian Mg2+-dependent phosphatidate phosphatase (PAP), is a protein that has dual functions as a PAP in the triglyceride synthesis pathway and as a transcriptional co-activator to promote fat oxidation and suppress de novo lipogenesis (62). The gene encoding lipin-1 (LPIN1) was originally identified though positional cloning of the mutant gene underlying lipodystrophy in the fatty liver dystrophy (fld) mouse (63). In the liver, two major lipin-1 protein isoforms (both containing a putative nuclear localization signal) are generated through alternative mRNA splicing of an internal exon within the LPIN1, namely, lipin-1α (891 amino acids), and lipin-1β (924 amino acids) (64).
In cultured hepatocytes, lipin-1α is predominantly located in the nucleus, whereas lipin-1β is primarily located in the cytoplasm (64). In the cytoplasm, lipin-1β-mediated PAP enzyme converts phosphatidate (PA) to diacylglycerol (DAG). The resulting DAG serves as substrate for the synthesis of TG as well as phosphatidylcholine (PC) and phosphatidylethanolamine (PE) (64). The lipin-1β is also associated with increased expression of lipogenic genes, and excessive hepatic fat accumulation in animals (64). In the nucleus, lipin-1α protein directly interacts with several nuclear receptors such as PGC-1α, PPARα and SREBP-1 and functions as a transcriptional co-activator to either stimulate the expression of mitochondrial genes involved in fatty acid oxidation or suppress the expression of lipogenic genes involved in lipogenesis (65-67). A splicing factor, namely, SFRS10 (official gene name, TRA2B), emerges as an important modulator of alternative splicing of LPIN1 (68,69). The effects of reduced SFRS10 on LPIN1 splicing, thereby favoring the LPIN1β isoform, have been shown to be sufficient to increase expression of lipogenic genes, activate lipogenesis, and cause excessive fat accumulation in the livers of high-fat-fed mice and in obese humans (68).
Ethanol-mediated dysregulation of hepatic lipin-1 function contributes to the abnormalities in hepatic lipid metabolism associated with AFLD (13,17,19,20,27,54,65,66,70). The development of AFLD in rodents and in humans is associated with significantly increased total hepatic lipin-1 gene expression and lipin-1-mediated PAP activity (27,65,66,70). More importantly, while ethanol induces the cytoplasmic pro-lipogenic activity of lipin-1, lipin-1 nuclear entry is attenuated by ethanol exposure in cultured hepatocytes and in mouse livers (27). The net consequence of these ethanol-mediated effects on lipin-1 can enhance de novo lipogenesis and inhibit fatty acid, ultimately leading to development of liver steatosis.
Intriguingly, the AMPK-SREBP axis is found to be involved in the regulation of total lipin-1 gene expression induced by ethanol (27,71). Additionally, ethanol feeding to mice significantly increased acetylation level of hepatic lipin-1, while at the same time markedly increased its SUMOylation levels (27). The SUMOylation of lipin-1α is needed for its nuclear localization and co-regulator activity toward PGC-1α (72). Attenuated lipin-1 nuclear entry in response to ethanol challenge may be mediated through disturbing the interplay between acetylation/SUMOylation modifications of lipin-1 that eventually disrupts lipin-1 signaling.
Interestingly, ethanol exposure also markedly elevated the ratio of hepatic Lpin1β/α via SFRS10 suppression in cultured hepatocytes and in mice (17,19,20). Consistently, hepatic SIRT1 deficiency significantly augmented the ethanol-mediated increase of Lpin1β/α via SFRS10, implying that ethanol-mediated SIRT1 inhibition might reduce hepatic SFRS10 in mice (20). Indeed, while incubation of AML-12 hepatocytes with ethanol increased intracellular lipid accumulation, this fat accumulation was largely reversed when AML-12 cells were transfected with SIRT1wt or SFRS10wt, via blocking the ability of ethanol to increase the ratio of Lpin1β/α (20). These findings clearly suggest a causal role of SIRT1-SFRS10-lipin-1 axis in the development of alcoholic steatosis in mice. Paralleling these findings in mice, similar changes in mRNA levels of SIRT1, lipin-1α or SFRS10 were all found in liver samples from patients with alcoholic hepatitis (20). The precise mechanism by which SIRT1 regulates SFRS10 gene and protein expression in the livers of ethanol-fed mice remains to be elucidated.
SIRT1-FoxO1 signaling and AFLD
FoxO1 has been established as a key player in the regulation of various pathways involved in lipid metabolism and oxidative stress response (73). FoxO1 activity is regulated by changes in its subcellular localization in association with its post-translational modifications, including acetylation/deacetylation (73,74). SIRT1 deacetylates the lysine residues within the FoxO1 DNA binding domain, and promotes nuclear retention of FoxO1 by either increasing or decreasing its transcriptional activity (73). Ethanol administration increases the acetylation of FoxO1, and subsequently downregulates nuclear FoxO1 protein in the livers of ethanol-fed mice (14,75). These observations clearly link an impaired liver SIRT1-FoxO1 axis with AFLD in mice.
Acute ethanol induces autophagy and partly reduces ethanol-induced liver injury (76). FoxO3a, another member of the FoxO family of transcription factors, is a vital molecule in regulating alcohol-induced autophagy and cell survival (76). Resveratrol-mediated SIRT1 activation further enhances ethanol-induced expression of autophagy-related genes, most likely by increasing deacetylation of FoxO3a, thus suggesting an involvement of SIRT1-FoxO3 axis in the ethanol action (76).
SIRT1-mediated histone H3 deacetylation and AFLD
SIRT1 can regulate gene expression by directly regulating histones. Although substrate specificity of SIRT1 has not been rigorously defined, mouse SIRT1 has been demonstrated to deacetylate histones with a preference for histone H3 at lysine 9 (Lys 9) and histone H4 at lysine 16 (Lys16) (77,78).
Chronic ethanol exposure has been shown to drastically and selectively increase acetylation of histone H3-Lys9 both in vitro and in animal liver (9,13,27,79-83). Conceivably, Histone H3-Lys9 deacetylation may occur at a significantly slower rate as a result of SIRT1 inhibition, thereby causing hyperacetylation of histone H3-Lys9 in the liver of ethanol-challenged animals. Intriguingly, activation of hepatic SREBP-1-lipin-1 axis by ethanol is also accompanied by its association with dramatically increased acetylated histone H3-lys9, suggesting that the SIRT1-histone H3 axis may regulate the expression of genes encoding lipogenic enzymes via SREBP-1-lipin-1 axis (72). Moreover, the protective effects of a diet high in saturated fatty acids against AFLD occur partially via the hepatic SIRT1-SREBP-1-histone H3-Lys9 axis, suppressing the expression of genes encoding lipogenic enzymes and slowing the synthesis of hepatic fatty acids (9).
It is worthwhile to note that SIRT1 directly deacetylates and represses the activity of acetyltransferase p300/CBP (84). Therefore, it is logical to speculate that ethanol may indirectly activate CBP/p300 through its inhibition on SIRT1, causing further hyperacetylation of various hepatic target proteins.
SIRT1-Wnt/β-catenin signaling and AFLD
Aberrant Wnt/β-catenin is a critical contributor to several forms of liver injury, including liver degeneration, liver cancer and alcoholic liver disease (16,85). The livers of acute ethanol-challenged β-catenin-deficient (β-catenin KO) mice displayed redox imbalance, impaired PPARα-mediated mitochondrial fatty acid oxidation and severe liver steatosis (16). Further mechanistic studies revealed, in the absence of β-catenin, there were significant decreases in SIRT1 mRNA and protein levels in the livers of β-catenin KO mice following ethanol administration (16). As discussed above, SIRT1 is an established regulator of PPARα function and mitochondrial fatty acid oxidation. Therefore, hepatic Wnt/β-catenin signaling is likely associated with AFLD via modifying the SIRT1-PPARα signaling axis.
SIRT1-NF-κB axis and ethanol-mediated hepatic inflammation
SIRT1 exerts anti-inflammatory effects by deacetylation of the lysine residues on NF-κB, which is a master transcription factor involved in regulation of pro-inflammatory cytokines (1,55,86). Ethanol-mediated hepatic inflammatory response has been linked to elevated levels of gut-derived lipopolysaccharide (LPS), a major component of bacterial cell walls, and generation of major ethanol metabolites such as acetaldehyde and acetate (6). LPS or ethanol metabolites-induced overproduction of pro-inflammatory cytokines, such as tumor necrosis factor α (TNF-α), is a major contributor to the pathogenesis of alcoholic liver disease (6). Interestingly, LPS, acetaldehyde, or acetate significantly inhibited SIRT1 in cultured rat Kupffer cells and murine RAW 264.7 macrophages (11). Inhibition of SIRT1 by three putative agents of ALD resulted in a significant increase in the TNF-α generation, largely through disrupting SIRT1-mediated deacetylation of NF-κB in cultured macrophages (11). Consistently, ethanol administration exacerbated the inflammatory response in the livers of Sirt1LKO mice, as indicated by substantially enhanced levels of F4/80+ staining accompanied by elevated mRNA expression levels of pro-inflammatory cytokines, including TNF-α and monocyte chemoattractant protein 1 (20).
NFATc4 is a non-nuclear receptor transcription factor involved in the regulation of pro-inflammatory cytokines such as TNF-α or IL-6 (20,53,87). NFATc4 nuclear localization and activity is generally regulated by calcineurin-mediated phosphorylation/de-phosphorylation. Ethanol administration to Sirt1LKO robustly promotes nuclear accumulation and activation of NFATc4, suggesting that SIRT1 acts as a repressor of NFATc4 activity (20). However, it remains unknown whether NFATc4 activity can be regulated by SIRT1 via acetylation/de-acetylation.
Recent evidence demonstrates that lipin-1 has potent anti-inflammatory properties (20,53,87). Lipin-1 suppressed expression of pro-inflammatory cytokines such as TNF-α by directly interaction with NFATc4 to inhibit its activity in adipocytes (87). Interestingly, liver lipin-1 ablation markedly increased the expression of pro-inflammatory cytokines via activation of both NFATc4 and NF-κB in mice fed with or without ethanol, implying a considerable interplay among NFATc4, NF-κB, and lipin-1 (53). Taken together, our laboratory has focused our attention in investigating the relationship among SIRT1, NF-κB, or NFATc4 and how SIRT1-NF-κB/NFATc4 axis is regulated by ethanol in Kupffer cells.
Adiponectin-SIRT1 axis and AFLD
Hypoadiponectinemia and altered hepatic adiponectin signaling are associated with steatosis and inflammation in several animal ALFD models such as mice, rats and micropigs (55). Stimulation of adipocytes adiponectin and hepatic adiponectin receptor (AdipoR)-mediated signaling by dietary or pharmacological manipulation largely alleviates development of AFLD in animals (55). Despite the exact mechanisms whereby ethanol affects the expression, production and secretion of adiponectin in adipose tissue remain to be elucidated, hepatic SIRT1 has emerged as one of the major mediators for adiponectin’s beneficial effects on AFLD. Adipose adiponectin gene and protein expression are up-regulated by PPARγ, of which rosiglitazone is a potent agonist (13,54). Co-administration of rosiglitazone and ethanol to mice significantly increased the gene expression and circulating levels of total adiponectin and its high-molecular-weight (HMW) form (13). More importantly, these increases correlated closely with the activation of hepatic SIRT1 signaling and prevention of fatty liver in mice (13). Similarly, the resveratrol-mediated elevation of circulating adiponectin levels was associated with robust enhancement of hepatic SIRT1 protein expression and attenuation of hepatic lipid accumulation in chronically ethanol-fed mice (10). Those findings suggest that stimulated hepatic adiponectin-SIRT1 signaling partially contributed to the protective action of both rosiglitazone and resveratrol against AFLD in mice. It is important to point out that it is unlikely that the effects of ethanol on SIRT1 are mediated entirely via disrupting adiponectin signaling, as inhibitory effects of ethanol on SIRT1 are also observed in cultured hepatocytes, which would not be expected to be influenced by adiponectin.
Ethanol down-regulates hepatic SIRT1
Ethanol exposure (chronic, acute or chronic-binge) reduces SIRT1 gene and protein expression levels, induces SIRT1 nucleocytoplasmic shuttling, and ultimately inhibits SIRT1 deacetylase activity in the liver (7-21) (Figure 2). As we have discussed above, while the role of SIRT1 signaling in the development of AFLD is firmly established, yet the regulatory mechanisms of hepatic SIRT1 expression and activity in response to ethanol challenge needs to be fully elucidated.
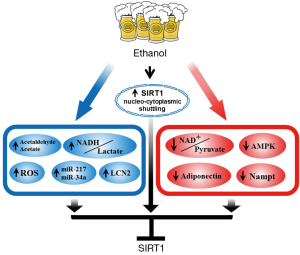
Ethanol metabolism
Ethanol is mainly metabolized in the liver by class I (low Km) alcohol dehydrogenase (ADH) and aldehyde dehydrogenase 2 (ALDH2) enzymes, which in turn shifts the redox state, resulting in accumulation of NADH, reduction of NAD+, a significant shift in (NAD+)/(NADH), and elevation of the lactate/pyruvate ratio (6). Cellular redox status regulates SIRT1 expression and activity (5). Logically, ethanol metabolism-mediated redox shift favoring NADH could inhibit SIRT1 activity in liver. Moreover, in cultured macrophage cell lines, two major ethanol metabolites, acetaldehyde and acetate, also significant inhibit SIRT1 expression and activity (11).
Nicotinamide phosphoribosyltransferase (NAMPT) is a key enzyme in the salvaging pathway for the synthesis of NAD+ (88). The enzymatic activity of NAMPT also directly reduces cellular NAM levels (89). Both of these actions of NAMPT can alter SIRT1 activity. The aberrant NAMPT signaling in the development of NAFLD has been demonstrated (90-92). Ethanol exposure may also compromise the NAMPT-mediated NAD+ biosynthesis, leading to SIRT1 inhibition. Nevertheless, the mechanism of ethanol disruption of the NAMPT-mediated NAD+ salvage pathway and subsequent inhibition of SIRT1 deacetylase activity will need to be further investigated.
Reactive oxygen species (ROS)
ROS inhibit SIRT1 activity and disrupt SIRT1 signaling (1,5). Overwhelming evidences indicate that oxidative stress contributes to alcoholic liver injury (6,91). Ethanol metabolism-generated oxidative stress has been proposed as a crucial contributor to the “second hit” that turned a simple liver steatosis into advanced stage liver injuries. ROS are generated via multiple pathways in the hepatic ethanol metabolism, particularly the CYP2E1 microsomal ethanol oxidizing pathway. Ethanol metabolism-induced ROS production could be an important causative factor for the inhibition of SIRT1 by ethanol. It is also tempting to postulate that ethanol-mediated ROS production may also prolong the suppression of SIRT1 activity in the liver as the rodents adapted to chronic ethanol exposure.
Hepatic SIRT1 ablation induces oxidative stress, as demonstrated by an increase in hepatic malondialdehyde, a lipid peroxidation end product, in mice (20). The oxidative stress is further drastically exacerbated in response to ethanol administration in Sirt1LKO mice, suggesting that ethanol-mediated down regulation of SIRT1 may serve as a feedback loop to further inhibit SIRT1 via ROS generation and diminish the protective capacity of liver against oxidative stress, increasing the sensitivity of liver to stress damage (20).
The ability of LPS, acetaldehyde, or acetate to induce ROS has been demonstrated in cultured hepatic cells (11). Thus, the putative agents of AFLD may alter signaling cascades such as oxidative stress signaling that ultimately impinge on hepatic SIRT1 (11). Moreover, ethanol metabolism-induced reduction of SIRT1 activity may increase the vulnerability of liver to insults (second “hit”) such as portal vein LPS or TNF-α, leading to the development of severe liver injuries.
miRNAs
A growing body of evidence suggests that aberrant expression of hepatic microRNAs (miRNAs) contributes to the development of alcoholic liver injury (92). Thus, miRNAs have been suggested as potential novel therapeutic targets for AFLD.
Recently, we have discovered that ethanol exposure drastically and specifically induced a known endogenous SIRT1 inhibitor, namely, miR-217, in AML-12 hepatocytes and in mouse livers (17). Overexpression of miR-217 in AML-12 hepatocytes promoted ethanol-mediated impairments of SIRT1 activity and SIRT1-regulated genes encoding lipogenic and fatty acid oxidation enzymes, and exacerbated alcoholic steatosis (17). Aberrant expression of miR-34a, one of known SIRT1 inhibitors, occurs in the processes that contribute to ALD progression. miR-34a is significantly elevated in ethanol-exposed hepatobiliary cell lines and in the livers of mice fed with ethanol, largely via SIRT1 inhibition (93).
There are other miRs known to regulate SIRT1 in addition to miR-217 and miR-34a (94). Their involvement in the development of AFLD will need to be further investigated. Moreover, the in vivo causal evidence of these miRs awaits confirmation utilizing the gain and/or loss of function approaches in mice.
Ethanol induces SIRT1 nucleocytoplasmic shuttling
SIRT1 is predominately located in the nucleus. SIRT1 activity is regulated by nucleocytoplasmic shuttling (95,96). A number of stimuli such as ROS lead to the translocation of SIRT1 from the nucleus, and disturbs SIRT1 activity (95-97). Ethanol exposure induced transport of SIRT1 from the nucleus to the cytoplasm in either cultured hepatocytes or in animal liver (14,17,19). Pre-treatment with an antioxidant, N-acetylcystine, largely prevented the ethanol-induced shuttling of SIRT1 to the cytoplasm, suggesting that ethanol-induced shuttling of SIRT1 is likely redox dependent (14).
Adiponectin
Adiponectin directly up-regulates SIRT1 in cultured hepatic cells. In cultured macrophages, treatment with mammalian globular adiponectin significantly increased SIRT1 protein levels in a dose-dependent manner (11). Although SIRT1 protein levels were significantly reduced by treatment with LPS or acetate, pre-incubation with globular adiponectin largely relieved LPS- or acetate-induced SIRT1 inhibition in cultured macrophages or Kupffer cells.
Consistently, in rat H4IIEC3 cells, full-length adiponectin significantly increased SIRT1 protein levels in a dose dependent manner (13). Knocking down both AdipoRs 1 and 2 (AdipoR1/2) completely abolished the elevation of SIRT1 by adiponectin, indicating direct involvement of adiponectin in the process (13). Moreover, the inhibition of SIRT1 by ethanol in H4IIEC3 cells was largely alleviated by treatment of adiponectin (13).
Lipocalin 2 (Lcn2)
Lcn2 [also named as SIP24/24p3 in mouse and neutrophil gelatinase-associated lipocalin (NGAL) in human], is a 25-kDa secretory small glycoprotein (98). We have recently discovered that ethanol administration elicited Lcn2 gene up-regulation and its protein expression in the liver, which led to elevated circulating Lcn2 levels and development of AFLD in mice. Interestingly, adenovirus-mediated overexpression of Lcn2 in the hepatocytes or mouse liver deteriorated to AFLD in correlation with inhibited SIRT1 protein expression (99).
Summary and conclusions
In recent years, SIRT1 has been gaining recognition as a central regulator signaling molecule in the pathogenesis of AFLD (7-21). Ethanol-caused SIRT1 dysfunction in the liver leads to development of steatosis and inflammation via disrupting multiple regulatory pathways of lipid metabolism and hepatocellular inflammatory processes through impairment of a signaling network involved by various vital transcriptional regulators and co-regulators as well as histones in rodents and humans (Figures 1,2).
Ample evidence demonstrates that ethanol has inhibitory effects on SIRT1 expression and on SIRT-mediated deacetylase activity (7-21). However, the precise regulatory mechanism by which ethanol inhibits SIRT1 activity and compromises SIRT1 signaling will require further elucidation. Given that SIRT1 can be regulated at multiple levels including NAD+ levels and posttranslational modifications, it will be of great importance to examine whether and how ethanol affects acetylation, phosphorylation and SUMOylation of SIRT1, which can eventually impair SIRT1 activity. Moreover, SIRT1 activity can be modified by its interaction with other proteins. For example, DBC1 (deleted in breast cancer 1) has been identified as a negative regulator of SIRT1 (100). It would be interesting to explore the effects of ethanol on the interaction of SIRT1 and DBC1, and its relationship with AFLD. Additionally, deregulation of the intracellular localization of SIRT1 by ethanol warrants additional investigations. Exactly how ethanol interferences with the diverse regulatory mechanisms that fine-tune the activity of hepatic SIRT1 will needs to be further studied.
Further studies will be necessary to clarify the effects of ethanol or its relationship with other crucial machineries in the liver on the dynamics of the SIRT1-driven signaling regulatory networks, which ultimately cause AFLD. SIRT1 plays a pivotal role in regulating several newly emerged machineries such as circadian rhythms and autophagy (1-3). Impaired circadian rhythms and disturbed autophagy are associated with alcoholic liver injury (76,101). Therefore, it will be important to determine whether and how ethanol-mediated SIRT1 inhibition links to impairment of hepatocellular processes such as circadian rhythms or autophagy and subsequently contributes to pathogenesis of AFLD. Considerable evidences indicate mammalian target of rapamycin complex 1 (mTORC1) as a vital and central upstream regulator of SIRT1 (1-3). Ethanol activates hepatic mTORC1 activity in animal liver (19). It will be worthwhile to further characterize the functional role of mTORC1 in the development of AFLD.
In addition to SIRT1, other sirtuin family members (e.g., SIRT2-7) also play important roles in the development of AFLD (4). For example, the functions of mitochondrial sirtuins (e.g., SIRT3-5) may be compromised by ethanol, potentially resulting in the mitochondrial dysfunction observed in AFLD (6,102,103). It will be importance to investigate the interplay between SIRT1 and SIRT2-7 in the liver and how the coordination of sirtuin family member’s network is disrupted by ethanol.
Finally, studies on clinical patients with AFLD are needed to translate our knowledge on SIRT1 in AFLD to human health. Undoubtedly, continued search for effective pharmacological and/or nutritional reagents for promoting hepatic SIRT1 activity or optimizing SIRT1 signaling will ultimately provide novel and effective remedies for human AFLD.
Acknowledgements
This study was supported by grants from National Institute on Alcoholism and Alcohol Abuse (AA013623 and AA015951 to Min You, PhD).
Disclosure: The authors declare no conflict of interest.
References
- Schug TT, Li X. Sirtuin 1 in lipid metabolism and obesity. Ann Med 2011;43:198-211. [PubMed]
- Chang HC, Guarente L. SIRT1 and other sirtuins in metabolism. Trends Endocrinol Metab 2014;25:138-45. [PubMed]
- Wang S, Moustaid-Moussa N, Chen L, et al. Novel insights of dietary polyphenols and obesity. J Nutr Biochem 2014;25:1-18. [PubMed]
- Kitada M, Kume S, Kanasaki K, et al. Sirtuins as possible drug targets in type 2 diabetes. Curr Drug Targets 2013;14:622-36. [PubMed]
- Revollo JR, Li X. The ways and means that fine tune Sirt1 activity. Trends Biochem Sci 2013;38:160-7. [PubMed]
- Gao B, Bataller R. Alcoholic liver disease: pathogenesis and new therapeutic targets. Gastroenterology 2011;141:1572-85. [PubMed]
- You M, Liang X, Ajmo JM, et al. Involvement of mammalian sirtuin 1 in the action of ethanol in the liver. Am J Physiol Gastrointest Liver Physiol 2008;294:G892-8. [PubMed]
- Lieber CS, Leo MA, Wang X, et al. Effect of chronic alcohol consumption on Hepatic SIRT1 and PGC-1alpha in rats. Biochem Biophys Res Commun 2008;370:44-8. [PubMed]
- You M, Cao Q, Liang X, et al. Mammalian sirtuin 1 is involved in the protective action of dietary saturated fat against alcoholic fatty liver in mice. J Nutr 2008;138:497-501. [PubMed]
- Ajmo JM, Liang X, Rogers CQ, et al. Resveratrol alleviates alcoholic fatty liver in mice. Am J Physiol Gastrointest Liver Physiol 2008;295:G833-42. [PubMed]
- Shen Z, Ajmo JM, Rogers CQ, et al. Role of SIRT1 in regulation of LPS- or two ethanol metabolites-induced TNF-alpha production in cultured macrophage cell lines. Am J Physiol Gastrointest Liver Physiol 2009;296:G1047-53. [PubMed]
- Qin Y, Tian YP. Exploring the molecular mechanisms underlying the potentiation of exogenous growth hormone on alcohol-induced fatty liver diseases in mice. J Transl Med 2010;8:120. [PubMed]
- Shen Z, Liang X, Rogers CQ, et al. Involvement of adiponectin-SIRT1-AMPK signaling in the protective action of rosiglitazone against alcoholic fatty liver in mice. Am J Physiol Gastrointest Liver Physiol 2010;298:G364-74. [PubMed]
- Liang X, Hu M, Rogers CQ, et al. Role of SIRT1-FoxO1 signaling in dietary saturated fat-dependent upregulation of liver adiponectin receptor 2 in ethanol-administered mice. Antioxid Redox Signal 2011;15:425-35. [PubMed]
- Leung TM, Lu Y, Yan W, et al. Argininosuccinate synthase conditions the response to acute and chronic ethanol-induced liver injury in mice. Hepatology 2012;55:1596-609. [PubMed]
- Lehwald N, Tao GZ, Jang KY, et al. β-Catenin regulates hepatic mitochondrial function and energy balance in mice. Gastroenterology 2012;143:754-64. [PubMed]
- Yin H, Hu M, Zhang R, et al. MicroRNA-217 promotes ethanol-induced fat accumulation in hepatocytes by down-regulating SIRT1. J Biol Chem 2012;287:9817-26. [PubMed]
- Nascimento AF, Ip BC, Luvizotto RA, et al. Aggravation of nonalcoholic steatohepatitis by moderate alcohol consumption is associated with decreased SIRT1 activity in rats. Hepatobiliary Surg Nutr 2013;2:252-9. [PubMed]
- Everitt H, Hu M, Ajmo JM, et al. Ethanol administration exacerbates the abnormalities in hepatic lipid oxidation in genetically obese mice. Am J Physiol Gastrointest Liver Physiol 2013;304:G38-47. [PubMed]
- Yin H, Hu M, Liang X, et al. Deletion of SIRT1 from hepatocytes in mice disrupts lipin-1 signaling and aggravates alcoholic fatty liver. Gastroenterology 2014;146:801-11. [PubMed]
- Li M, Lu Y, Hu Y, et al. Salvianolic acid B protects against acute ethanol-induced liver injury through SIRT1-mediated deacetylation of p53 in rats. Toxicol Lett 2014;228:67-74. [PubMed]
- Hubbard BP, Gomes AP, Dai H, et al. Evidence for a common mechanism of SIRT1 regulation by allosteric activators. Science 2013;339:1216-9. [PubMed]
- You M, Matsumoto M, Pacold CM, et al. The role of AMP-activated protein kinase in the action of ethanol in the liver. Gastroenterology 2004;127:1798-808. [PubMed]
- Tomita K, Tamiya G, Ando S, et al. AICAR, an AMPK activator, has protective effects on alcohol-induced fatty liver in rats. Alcohol Clin Exp Res 2005;29:240S-5S. [PubMed]
- Esfandiari F, You M, Villanueva JA, et al. S-adenosylmethionine attenuates hepatic lipid synthesis in micropigs fed ethanol with a folate-deficient diet. Alcohol Clin Exp Res 2007;31:1231-9. [PubMed]
- Xu J, Lai KK, Verlinsky A, et al. Synergistic steatohepatitis by moderate obesity and alcohol in mice despite increased adiponectin and p-AMPK. J Hepatol 2011;55:673-82. [PubMed]
- Hu M, Wang F, Li X, et al. Regulation of hepatic lipin-1 by ethanol: role of AMP-activated protein kinase/sterol regulatory element-binding protein 1 signaling in mice. Hepatology 2012;55:437-46. [PubMed]
- Shearn CT, Smathers RL, Jiang H, et al. Increased dietary fat contributes to dysregulation of the LKB1/AMPK pathway and increased damage in a mouse model of early-stage ethanol-mediated steatosis. J Nutr Biochem 2013;24:1436-45. [PubMed]
- Lee HI, McGregor RA, Choi MS, et al. Low doses of curcumin protect alcohol-induced liver damage by modulation of the alcohol metabolic pathway, CYP2E1 and AMPK. Life Sci 2013;93:693-9. [PubMed]
- Mandal S, Mukhopadhyay S, Bandhopadhyay S, et al. 14-Deoxyandrographolide alleviates ethanol-induced hepatosteatosis through stimulation of AMP-activated protein kinase activity in rats. Alcohol 2014;48:123-32. [PubMed]
- Lee HI, Yun KW, Seo KI, et al. Scopoletin prevents alcohol-induced hepatic lipid accumulation by modulating the AMPK-SREBP pathway in diet-induced obese mice. Metabolism 2014;63:593-601. [PubMed]
- Ruderman NB, Xu XJ, Nelson L, et al. AMPK and SIRT1: a long-standing partnership? Am J Physiol Endocrinol Metab 2010;298:E751-60. [PubMed]
- Lan F, Cacicedo JM, Ruderman N, et al. SIRT1 modulation of the acetylation status, cytosolic localization, and activity of LKB1. Possible role in AMP-activated protein kinase activation. J Biol Chem 2008;283:27628-35. [PubMed]
- Hou X, Xu S, Maitland-Toolan KA, et al. SIRT1 regulates hepatocyte lipid metabolism through activating AMP-activated protein kinase. J Biol Chem 2008;283:20015-26. [PubMed]
- Zhang HS, Wu MR. SIRT1 regulates Tat-induced HIV-1 transactivation through activating AMP-activated protein kinase. Virus Res 2009;146:51-7. [PubMed]
- Cantó C, Gerhart-Hines Z, Feige JN, et al. AMPK regulates energy expenditure by modulating NAD+ metabolism and SIRT1 activity. Nature 2009;458:1056-60. [PubMed]
- You M, Fischer M, Deeg MA, et al. Ethanol induces fatty acid synthesis pathways by activation of sterol regulatory element-binding protein (SREBP). J Biol Chem 2002;277:29342-7. [PubMed]
- Ji C, Kaplowitz N. Betaine decreases hyperhomocysteinemia, endoplasmic reticulum stress, and liver injury in alcohol-fed mice. Gastroenterology 2003;124:1488-99. [PubMed]
- Tomita K, Azuma T, Kitamura N, et al. Pioglitazone prevents alcohol-induced fatty liver in rats through up-regulation of c-Met. Gastroenterology 2004;126:873-85. [PubMed]
- Esfandiari F, Villanueva JA, Wong DH, et al. Chronic ethanol feeding and folate deficiency activate hepatic endoplasmic reticulum stress pathway in micropigs. Am J Physiol Gastrointest Liver Physiol 2005;289:G54-63. [PubMed]
- Ji C, Chan C, Kaplowitz N. Predominant role of sterol response element binding proteins (SREBP) lipogenic pathways in hepatic steatosis in the murine intragastric ethanol feeding model. J Hepatol 2006;45:717-24. [PubMed]
- Pastorino JG, Shulga N. Tumor necrosis factor-alpha can provoke cleavage and activation of sterol regulatory element-binding protein in ethanol-exposed cells via a caspase-dependent pathway that is cholesterol insensitive. J Biol Chem 2008;283:25638-49. [PubMed]
- Passeri MJ, Cinaroglu A, Gao C, et al. Hepatic steatosis in response to acute alcohol exposure in zebrafish requires sterol regulatory element binding protein activation. Hepatology 2009;49:443-52. [PubMed]
- Ambade A, Catalano D, Lim A, et al. Inhibition of heat shock protein 90 alleviates steatosis and macrophage activation in murine alcoholic liver injury. J Hepatol 2014;61:903-11. [PubMed]
- He L, Simmen FA, Ronis MJ, et al. Post-transcriptional regulation of sterol regulatory element-binding protein-1 by ethanol induces class I alcohol dehydrogenase in rat liver. J Biol Chem 2004;279:28113-21. [PubMed]
- He L, Simmen FA, Mehendale HM, et al. Chronic ethanol intake impairs insulin signaling in rats by disrupting Akt association with the cell membrane. Role of TRB3 in inhibition of Akt/protein kinase B activation. J Biol Chem 2006;281:11126-34. [PubMed]
- Ponugoti B, Kim DH, Xiao Z, et al. SIRT1 deacetylates and inhibits SREBP-1C activity in regulation of hepatic lipid metabolism. J Biol Chem 2010;285:33959-70. [PubMed]
- Walker AK, Yang F, Jiang K, et al. Conserved role of SIRT1 orthologs in fasting-dependent inhibition of the lipid/cholesterol regulator SREBP. Genes Dev 2010;24:1403-17. [PubMed]
- Nemoto S, Fergusson MM, Finkel T. SIRT1 functionally interacts with the metabolic regulator and transcriptional coactivator PGC-1{alpha}. J Biol Chem 2005;280:16456-60. [PubMed]
- Rodgers JT, Lerin C, Gerhart-Hines Z, et al. Metabolic adaptations through the PGC-1 alpha and SIRT1 pathways. FEBS Lett 2008;582:46-53. [PubMed]
- You M, Considine RV, Leone TC, et al. Role of adiponectin in the protective action of dietary saturated fat against alcoholic fatty liver in mice. Hepatology 2005;42:568-77. [PubMed]
- Zeng T, Zhang CL, Song FY, et al. CMZ reversed chronic ethanol-induced disturbance of PPAR-alpha possibly by suppressing oxidative stress and PGC-1alpha acetylation, and activating the MAPK and GSK3beta pathway. PLoS One 2014;9:e98658. [PubMed]
- Hu M, Yin H, Mitra MS, et al. Hepatic-specific lipin-1 deficiency exacerbates experimental alcohol-induced steatohepatitis in mice. Hepatology 2013;58:1953-63. [PubMed]
- You M, Rogers CQ. Adiponectin: a key adipokine in alcoholic fatty liver. Exp Biol Med (Maywood) 2009;234:850-9. [PubMed]
- Purushotham A, Schug TT, Xu Q, et al. Hepatocyte-specific deletion of SIRT1 alters fatty acid metabolism and results in hepatic steatosis and inflammation. Cell Metab 2009;9:327-38. [PubMed]
- Fischer M, You M, Matsumoto M, et al. Peroxisome proliferator-activated receptor alpha (PPARalpha) agonist treatment reverses PPARalpha dysfunction and abnormalities in hepatic lipid metabolism in ethanol-fed mice. J Biol Chem 2003;278:27997-8004. [PubMed]
- Hong F, Radaeva S, Pan HN, et al. Interleukin 6 alleviates hepatic steatosis and ischemia/reperfusion injury in mice with fatty liver disease. Hepatology 2004;40:933-41. [PubMed]
- Nakajima T, Kamijo Y, Tanaka N, et al. Peroxisome proliferator-activated receptor alpha protects against alcohol-induced liver damage. Hepatology 2004;40:972-80. [PubMed]
- Kong L, Ren W, Li W, et al. Activation of peroxisome proliferator activated receptor alpha ameliorates ethanol induced steatohepatitis in mice. Lipids Health Dis 2011;10:246. [PubMed]
- Tan X, Sun X, Li Q, et al. Leptin deficiency contributes to the pathogenesis of alcoholic fatty liver disease in mice. Am J Pathol 2012;181:1279-86. [PubMed]
- Zeng T, Zhang CL, Song FY, et al. Garlic oil alleviated ethanol-induced fat accumulation via modulation of SREBP-1, PPAR-α, and CYP2E1. Food Chem Toxicol 2012;50:485-91. [PubMed]
- Harris TE, Finck BN. Dual function lipin proteins and glycerolipid metabolism. Trends Endocrinol Metab 2011;22:226-33. [PubMed]
- Péterfy M, Phan J, Xu P, et al. Lipodystrophy in the fld mouse results from mutation of a new gene encoding a nuclear protein, lipin. Nat Genet 2001;27:121-4. [PubMed]
- Manmontri B, Sariahmetoglu M, Donkor J, et al. Glucocorticoids and cyclic AMP selectively increase hepatic lipin-1 expression, and insulin acts antagonistically. J Lipid Res 2008;49:1056-67. [PubMed]
- Brindley DN, Cooling J, Burditt SL, et al. The involvement of glucocorticoids in regulating the activity of phosphatidate phosphohydrolase and the synthesis of triacylglycerols in the liver. Effects of feeding rats with glucose, sorbitol, fructose, glycerol and ethanol. Biochem J 1979;180:195-9. [PubMed]
- Savolainen MJ, Baraona E, Pikkarainen P, et al. Hepatic triacylglycerol synthesizing activity during progression of alcoholic liver injury in the baboon. J Lipid Res 1984;25:813-20. [PubMed]
- Finck BN, Gropler MC, Chen Z, et al. Lipin 1 is an inducible amplifier of the hepatic PGC-1alpha/PPARalpha regulatory pathway. Cell Metab 2006;4:199-210. [PubMed]
- Pihlajamäki J, Lerin C, Itkonen P, et al. Expression of the splicing factor gene SFRS10 is reduced in human obesity and contributes to enhanced lipogenesis. Cell Metab 2011;14:208-18. [PubMed]
- Peterson TR, Sengupta SS, Harris TE, et al. mTOR complex 1 regulates lipin 1 localization to control the SREBP pathway. Cell 2011;146:408-20. [PubMed]
- Simpson KJ, Venkatesan S, Martin A, et al. Activity and subcellular distribution of phosphatidate phosphohydrolase (EC 3.1.3.4) in alcoholic liver disease. Alcohol Alcohol 1995;30:31-6. [PubMed]
- Ishimoto K, Nakamura H, Tachibana K, et al. Sterol-mediated regulation of human lipin 1 gene expression in hepatoblastoma cells. J Biol Chem 2009;284:22195-205. [PubMed]
- Liu GH, Gerace L. Sumoylation regulates nuclear localization of lipin-1alpha in neuronal cells. PLoS One 2009;4:e7031. [PubMed]
- Lettieri Barbato D, Aquilano K, Ciriolo MR. FoxO1 at the nexus between fat catabolism and longevity pathways. Biochim Biophys Acta 2014;1841:1555-60.
- Sasaki T, Kitamura T. Roles of FoxO1 and Sirt1 in the central regulation of food intake. Endocr J 2010;57:939-46. [PubMed]
- Lieber CS, Leo MA, Wang X, et al. Alcohol alters hepatic FoxO1, p53, and mitochondrial SIRT5 deacetylation function. Biochem Biophys Res Commun 2008;373:246-52. [PubMed]
- Ni HM, Du K, You M, et al. Critical role of FoxO3a in alcohol-induced autophagy and hepatotoxicity. Am J Pathol 2013;183:1815-25. [PubMed]
- Vaquero A, Scher M, Lee D, et al. Human SirT1 interacts with histone H1 and promotes formation of facultative heterochromatin. Mol Cell 2004;16:93-105. [PubMed]
- Vaquero A, Scher MB, Lee DH, et al. SirT2 is a histone deacetylase with preference for histone H4 Lys 16 during mitosis. Genes Dev 2006;20:1256-61. [PubMed]
- Park PH, Miller R, Shukla SD. Acetylation of histone H3 at lysine 9 by ethanol in rat hepatocytes. Biochem Biophys Res Commun 2003;306:501-4. [PubMed]
- Park PH, Lim RW, Shukla SD. Involvement of histone acetyltransferase (HAT) in ethanol-induced acetylation of histone H3 in hepatocytes: potential mechanism for gene expression. Am J Physiol Gastrointest Liver Physiol 2005;289:G1124-36. [PubMed]
- Kim JS, Shukla SD. Histone h3 modifications in rat hepatic stellate cells by ethanol. Alcohol Alcohol 2005;40:367-72. [PubMed]
- Choudhury M, Park PH, Jackson D, et al. Evidence for the role of oxidative stress in the acetylation of histone H3 by ethanol in rat hepatocytes. Alcohol 2010;44:531-40. [PubMed]
- Park PH, Lim RW, Shukla SD. Gene-selective histone H3 acetylation in the absence of increase in global histone acetylation in liver of rats chronically fed alcohol. Alcohol Alcohol 2012;47:233-9. [PubMed]
- Bouras T, Fu M, Sauve AA, et al. SIRT1 deacetylation and repression of p300 involves lysine residues 1020/1024 within the cell cycle regulatory domain 1. J Biol Chem 2005;280:10264-76. [PubMed]
- Nejak-Bowen KN, Monga SP. Beta-catenin signaling, liver regeneration and hepatocellular cancer: sorting the good from the bad. Semin Cancer Biol 2011;21:44-58. [PubMed]
- Schug TT, Xu Q, Gao H, et al. Myeloid deletion of SIRT1 induces inflammatory signaling in response to environmental stress. Mol Cell Biol 2010;30:4712-21. [PubMed]
- Kim HB, Kumar A, Wang L, et al. Lipin 1 represses NFATc4 transcriptional activity in adipocytes to inhibit secretion of inflammatory factors. Mol Cell Biol 2010;30:3126-39. [PubMed]
- Dahl TB, Holm S, Aukrust P, et al. Visfatin/NAMPT: a multifaceted molecule with diverse roles in physiology and pathophysiology. Annu Rev Nutr 2012;32:229-43. [PubMed]
- Choi SE, Fu T, Seok S, et al. Elevated microRNA-34a in obesity reduces NAD+ levels and SIRT1 activity by directly targeting NAMPT. Aging Cell 2013;12:1062-72. [PubMed]
- Tao R, Wei D, Gao H, et al. Hepatic FoxOs regulate lipid metabolism via modulation of expression of the nicotinamide phosphoribosyltransferase gene. J Biol Chem 2011;286:14681-90. [PubMed]
- Sid B, Verrax J, Calderon PB. Role of oxidative stress in the pathogenesis of alcohol-induced liver disease. Free Radic Res 2013;47:894-904. [PubMed]
- McDaniel K, Herrera L, Zhou T, et al. The functional role of microRNAs in alcoholic liver injury. J Cell Mol Med 2014;18:197-207. [PubMed]
- Meng F, Glaser SS, Francis H, et al. Epigenetic regulation of miR-34a expression in alcoholic liver injury. Am J Pathol 2012;181:804-17. [PubMed]
- Choi SE, Kemper JK. Regulation of SIRT1 by microRNAs. Mol Cells 2013;36:385-92. [PubMed]
- Jin Q, Yan T, Ge X, et al. Cytoplasm-localized SIRT1 enhances apoptosis. J Cell Physiol 2007;213:88-97. [PubMed]
- Tanno M, Sakamoto J, Miura T, et al. Nucleocytoplasmic shuttling of the NAD+-dependent histone deacetylase SIRT1. J Biol Chem 2007;282:6823-32. [PubMed]
- Caito S, Rajendrasozhan S, Cook S, et al. SIRT1 is a redox-sensitive deacetylase that is post-translationally modified by oxidants and carbonyl stress. FASEB J 2010;24:3145-59. [PubMed]
- Wu G, Li H, Zhou M, et al. Mechanism and clinical evidence of lipocalin-2 and adipocyte fatty acid-binding protein linking obesity and atherosclerosis. Diabetes Metab Res Rev 2014;30:447-56. [PubMed]
- Jogasuria A, Gao B, You M. The Pivotal Role Played by Lipocalin-2 in Experimental Alcohol-Induced Fatty Liver Injury in Mice. Poster Sessions Hepatology 2014;60:92A-196A.
- Escande C, Chini CC, Nin V, et al. Deleted in breast cancer-1 regulates SIRT1 activity and contributes to high-fat diet-induced liver steatosis in mice. J Clin Invest 2010;120:545-58. [PubMed]
- Froy O. The relationship between nutrition and circadian rhythms in mammals. Front Neuroendocrinol 2007;28:61-71. [PubMed]
- Xue L, Xu F, Meng L, et al. Acetylation-dependent regulation of mitochondrial ALDH2 activation by SIRT3 mediates acute ethanol-induced eNOS activation. FEBS Lett 2012;586:137-42. [PubMed]
- Shulga N, Pastorino JG. Ethanol sensitizes mitochondria to the permeability transition by inhibiting deacetylation of cyclophilin-D mediated by sirtuin-3. J Cell Sci 2010;123:4117-27. [PubMed]