Extrahepatic organs in the development of non-alcoholic fatty liver disease in liver transplant patients
Introduction
Liver transplantation (LT) is the most effective therapeutic strategy for end-stage liver disease (1). Compared with other treatment options such as ablation or resection, LT takes its grips on higher long-term survival benefits (2). Non-alcoholic steatohepatitis (NASH), progressive form of non-alcoholic fatty liver disease (NAFLD), has become the fastest increasing indication and ranked second among all etiology for LT (3,4). Post-transplant NAFLD exhibits a relative high incidence with time-dependent characteristics in general. A systemic review reported the mean incidence of NAFLD after LT at 1-, 3-, 5-year were 59%, 57%, 82%, respectively (5). And this meta-analysis indicated NASH as pre-transplant etiology had higher prevalence of NASH after LT compared with other etiologies. Specifically, patients with NASH had mean 1-, 3- and 5-year post-transplant recurrent rates of 53%, 57.4%, 38%, respectively, but 13%, 16%, 17% for de novo NASH. Normally, steatosis is sackless in the absence of fibrosis in general population, but could deteriorate the state of illness and be at risk of developing post-transplant NAFLD/NASH with high prevalent metabolic syndrome and widely used immunosuppressant in LT cases (6-8).
LT offers a unique perspective to view hepatic and extrahepatic factors that define NAFLD with combination of donor graft and host factors. Genotypically, grafting of vitamin D receptor, VDR rs2228570, has lowers serum 25(OH)D, which influences NAFLD development (9,10). On the other side, graft PNPLA3 variant leads to liver steatosis phenotype and increases susceptibility to hepatic fat accumulation (11,12). Clinically, evidence shows that poor donor fat status increases the chances of NAFLD in graft recipients (13,14).
Whereas a lot is known the NAFLD in general population as well as graft-related factors in LT context, little is known about effects of extrahepatic factors on NAFLD in LT recipients. This review elucidates role of extrahepatic organs involved in NAFLD in LT cases context (Figure 1) and discuss current limitations in both preclinical and clinical scenarios with suggestions for future study. We present the following article in accordance with the Narrative Review reporting checklist (available at https://hbsn.amegroups.com/article/view/10.21037/hbsn-20-568/rc).
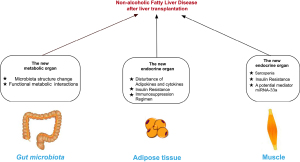
Methods
We searched the PubMed for the following keywords: NAFLD, NASH, liver transplant, therapy, pathogenesis and biomarkers. Only the published articles in English till 25th June 2020 in PubMed database were included and no limit was put on the study design type.
Gut disturbance promoting NAFLD post-transplantation
The gut is a bridge connecting the outer and inner environment with metabolites produced by microbiota. Recently, activities at the gut-liver axis have gained much attention in non-transplant patients (15). In LT background, it seems relative unique mechanisms of gut-liver communication involved in NAFLD.
LT, theoretically, could improve the structure of the gut microbiota because a functional liver graft could improve liver function and help maintain cell homeostasis. In their studies, Bajaj et al. confirmed the long-term benefits of LT in the microbiota composition and showed that there was restoration of microbiota in patients following LT (16). After 6 months of follow-up, the patients showed varying but significant improvements in the cognition abilities. In addition, whereas pathogenic microbiome or toxic endotoxins significantly reduced, probiotics increased confirmed by proteomic, lipidomic and metabolomic profiles.
On the contrary, a study that evaluated events in early post-transplant period found that the probiotics decreased while pathogenic microbiome increased (17). The decrease of beneficial bacteria such as Lactobacillus spp. and Bifidobacterium spp. might indicate a disturbance of intestinal barrier. Previous study showed elevated intestinal permeability by disrupting intestinal epithelial barrier and gut vascular barrier was early stage event engaged in NAFLD pathogenesis (18). Besides, studies profiled the similarity of gut microbiota between NAFLD and LT. For instance, Bifidobacterium is reduced in both NAFLD and in liver transplant cases (19,20). Xu et al. demonstrated a negative relevance of Bifidobacterium and hepatic total lipid content (r=−0.591, P=0.008) in mice. Supplementation with probiotics to restore intestinal homeostasis could significantly attenuate hepatic fat accumulation, thus may have potential association with NAFLD (21). Diet has a significant effect on the modulation of gut microbiota and therefore dietary modifications have a great potential to resolve NALFD. The proposed mechanism is that, the key components in the diet such as antioxidants and polyphenols, they interact with the gut microbiota and effect intestinal permeability and reduce endotoxemia (22-24). However, clinical trials on the use of probiotics and the specific diet to prevent NAFLD with comparative endpoints are unavailable in the context of LT (25-27). Future studies are needed to clarify their role.
Ischemia and microcirculatory dysfunction are common events during LT. It was reported that farnesoid X receptor (FXR) decreased dramatically in the ischemic/reperfusion injury phase (28). FXR, nuclear receptor of bile acids, is engaged in bile acid synthesis, lipid metabolism, energy expenditure, and inflammation (29). Animal experiment illustrated the mechanism of downregulation of FXR under hypoxia mediated by p38 mitogen-activated protein kinase (30), facilitating accumulation of intrahepatic bile acid. Previous study showed dysregulation of cytotoxic bile acid in NAFLD pathogenesis by interacted with FXR to modulate lipid metabolism throughout the gut-liver axis (31). Therefore, we assume a similar mechanism of gut dysbiosis mainly mediated by FXR contributing to NAFLD process after LT (Figures 2,3). Therapies targeting FXR have been developed to improve liver fat in clinical trials. The finished phase 2 clinical trial of obeticholic acid, a potent activator of FXR, has shown an improved liver histology defined by NAFLD activity score (50 out of 110, 45%) in obeticholic acid group compared with the counterpart in (23 out of 109, 21%) placebo group (relative risk, 2.2; 95% confidence interval, 1.4–3.3; P=0.0002) (29). A later phase 3 trial in the planed interim analysis reconfirmed the benefits of fibrosis improvement in 37 (12%) patients, 55 (18%), and 71 (23%) in the placebo group, obeticholic acid 10 mg group (P=0.045), obeticholic acid 25 mg group (P=0.0002), respectively (32). Although failing to meet the primary endpoint of NASH resolution, post hoc analysis showed attainment of secondary endpoint treated with obeticholic acid in NASH resolution without worsening of fibrosis based on pathologist diagnostic assessment. Additionally, it exhibited a tendency toward improvement in key components of NASH disease activity shown by decreased lobular inflammation or hepatocellular ballooning. Although the encouraging results of obeticholic acid for NAFLD therapy in clinical trial, it remains paucity in LT context to testify the benefits of FXR inhibitors. Therefore, further research and clinical trial are warrant to test the benefits of obeticholic acid in LT recipients.
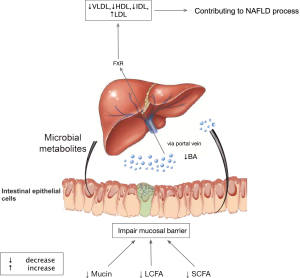
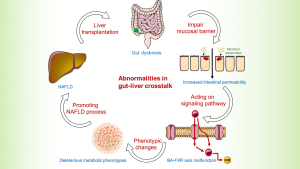
Adipose tissue disturbance promoting NAFLD post-transplantation
Post-transplant weight gain, obesity and hyperlipidemia are common after LT, irrespective of the etiology, indicating a disturbance of lipid metabolism (33-35). Obesity, mainly attributed to visceral adipose tissue, is a risk factor for NAFLD in general population (36). Recent studies showed relationship of NAFLD and obesity as well as other lipid-associated factors in LT background. A single-center retrospective study of LT recipients described the prevalence of de novo NAFLD was 36.1% of patients (37). Further multivariate analysis found predictive independent risk factors for de novo NAFLD 5-year after LT such as obesity (odds ratio, 3.72; 95% confidence interval, 1.27 to 10.90; P=0.017) and metabolic syndrome (odds ratio, 4.69; 95% confidence interval, 1.98 to l1.14; P<0.001) at fifth year post-LT. Another meta-analysis summarized seventeen studies representing 2,378 patients and found that post-transplant body mass index (BMI) (summarized odds ratio, 1.27; 95% confidence interval, 1.19–1.35) and post-transplant hyperlipidemia were independent promising predictors for NAFLD after LT, despite the conflict results of post-transplant hyperlipidemia significant in half researches (3/6 studies) (5).
Regarding adipose tissue as an endocrine organ provides a new perspective to understanding the pathogenesis of NAFLD in LT setting (38). There is a significant shift in adipokines level secreted by adipose tissue post-LT. Leptin, product of obesity gene, serves as hormone to modulate energy homeostasis and as cytokine to promote inflammatory responses (39). Animals study showed dual function of leptin in NAFLD pathogenesis with hepatic fat accumulation in leptin deficient circumstance and hepatic inflammation and fibrosis in leptin excess situation (40). Studies found leptin level decreased dramatically after LT (from 4.06 µg/L, 95% confidence interval, 3.45–5.68; to 3.32 µg/L, 95% confidence interval, 2.30–3.99; P=0.01) (41). Others reported an ascending trend of leptin by measuring different time points before transplantation and 4, 12, and 24 months after LT (42). Although animal confirmed mechanisms of leptin in NAFLD pathogenesis and definite association between circulating leptin and severity of NAFLD (40,43), there remains paucity of systemic review to clarify direct association of leptin and NAFLD under LT context. Intriguingly, a case reported leptin supplement for patient with recurrent NAFLD after LT greatly reduced hepatic fat accumulation supported by both histology and magnetic resonance imaging, which may serve as a promising therapy for such patient and need further clinical trials to confirm its effects (44). Cytokines are group of important mediators derived from adipocytes, immune system and endothelial cells. Recent studies support that the cytokine flare causes peripheral lipolysis, hepatic fat accumulation, inflammation, necrosis and fibrosis and hence are engaged in the process of NAFLD. Clinical studies have also shown a significant differential expression of TNF-α and IL-6 in NAFLD patients with respect to healthy controls (45-47). Previously NAFLD progression was thought to be tangled to type 1 inflammation originating in the adipose tissue. Previously NAFLD progression was thought to be tangled to type 1 inflammation originating in the adipose tissue. For instance, IL-1, which is an important proinflammatory cytokine and component of type 1 immunity, promotes local inflammation and limits adipose tissue expandability hence facilitating liver steatosis (48). However, Hart et al. demonstrated that TGF-β and IL-13 which were components of type 2 inflammation, exacerbated the progression of NAFLD in the liver and hence could be a therapeutic target for NASH (49). In LT background, an observational study showed similar inflammatory profiles between LT recipients and NASH patients compared with healthy controls after 1-year follow-up period (pg/mL): lower IL-10 levels (32.3 and 32.3 versus 62.5, respectively, P=0.019) and higher IFNγ (626.1 and 411.9 versus 67.9, respectively, P<0.001) (50). This may indicate a similar signaling pathway and therapeutic potential in LT and NASH. However, mechanistic studies deep into NAFLD/NASH in LT models are lacking thus need further efforts to work on.
Insulin resistance (IR) is engaged in NAFLD pathogenesis both in transplant and non-transplant settings (15,51). In LT context, the prevalence of immunosuppressant drugs is pivotal in systemic and local IR. Calcineurin inhibitors (CNIs), mainly tacrolimus and cyclosporine, have been shown in human adipocyte model to inhibit glucose uptake and increase IR by removing glucose transporter 4 from cell surface via elevated rate of endocytosis (52). Later work showed there may be other mechanisms besides endocytosis of CNIs on adipose tissue involving inhibition of glucose uptake mediated by gene transcription or protein translation, which needs more investigation (53). The second mechanism of CNIs on IR of adipose tissue is inhibiting protein phosphatases 2A, an independent activating way of phosphoinositide 3-kinase (54-56). Glucocorticoid (GC) is widely used in daily medical practice. Although GC is widely believed to cause a systemic IR, whether GC cause local IR in human adipose tissue is under debate. A randomized control study with 15 volunteers demonstrated that GC have no effect on insulin sensitivity of omental adipocyte (57). By contrast, later studies determined the role of FK506-binding protein 51 (FKBP51) and cannabinoid receptor 1 involved in glucocorticoid-induced IR in human adipose tissue (58,59). And mechanically, GC may contribute to adipose tissue IR via FKBP51-dependent regulation of AKT2-AS160 signaling (60). Another kind of immunosuppressive agents is mammalian target of rapamycin (mTOR) inhibitor. Insulin, most important signaling molecular, bridges IR and mTOR complex via phosphorylation of AKT1 (61). Animal mechanism study has demonstrated pathway involved in lipid homeostasis, termed mTOR/ERRα regulatory axis. Specifically, mTOR engaged in hepatic lipid accumulation by regulating ERRα via ubiquitin-mediated degradation through controlling of the ubiquitin-proteasome transcriptional expression (62).
Bariatric surgeries (BS), mainly gastric banding, sleeve gastrectomy and Roux-en-Y gastric bypass, are efficient to remove abdominal fat providing sustained benefits of weight loss as well as reducing incidence of NASH or HCC (63,64). Theoretically, pre-LT BS could prevent progression of NAFLD thus abrogating the need for LT. BS simultaneous with LT or post-LT may improve obesity-associated conditions and reduce the incidence of NAFLD in post-LT setting. In LT background, the ideal timing of BS has not been established as no studies providing direct comparators. And the exact efficacy of BS is controversy. A literature review showed that BS before, during, and after LT decreased the incidence of NAFLD with low to moderate evidence (65). However, another intention-to-treat analysis indicated prior BS was associated with lower 3-year survival rate compared with concurrent cohort from the time of listing (72% versus 82%; 95% confidence index, 59–87% versus 73–91.7%, respectively; P=0.055) (66). Recently, minimally invasive sleeve gastrectomy has been reported successfully performed in two LT recipients with condition of both obesity and recurrent or de novo NAFLD (67). Two patients showed improvement of steatosis and fibrosis status at discharge. To be specific, mean reduction of steatosis score was 0.34 (decrease from 0.98 to 0.74, 0.89 to 0.45, separately), and mean reduction of fibrosis score was 0.05 (decrease from 0.12 to 0.04, 0.16 to 0.14, separately), which need further validation in large cohort to confirm the benefits of reduction of adipose tissue and resolution of NAFLD.
Muscle disturbance promoting NAFLD post-transplantation
Sarcopenia is an age-related decrease in muscle mass, progressive loss of muscle strength and poor physical performance. Definition of sarcopenia varies among professional societies and organizations around the world (68-70). Recently, evidence-based literature searches have been performed by North American Working Group on Sarcopenia in Liver Transplantation to push forward the definition of sarcopenia in LT setting (71). It is preferred that sarcopenia is defined by skeletal muscle index (SMI) measured at L3 on abdominal computed tomography scan using sex-based cut-off values (SMI <50 cm2/m2 in men; SMI <39 cm2/m2 in women). And elements of sex, age and ethnicity should be considered to improve definition of sarcopenia in clinical practice.
Sarcopenia is increasingly recognized both in general population with liver disease and transplant recipients. Growing evidence has shown that sarcopenia is an emerging and independent risk factor for NAFLD in general population (72-74). Clinically, a recent systemic review including a total of 19 studies with 17 cross-sectional design and 2 retrospective cohorts evaluated the association of sarcopenia with NAFLD (75). Results demonstrated that patients with sarcopenia had significantly higher occurrence risks of NAFLD (odds ratio, 1.33; 95% confidence interval, 1.20–1.48) and NASH (odds ratio, 2.42; 95% confidence interval, 1.27–3.57). Mechanically, the pathogenesis of sarcopenia overlaps with NAFLD including IR, chronic low-grade inflammation, myokines, adipokines and physical inactivity (76).
Sarcopenia is a common event in transplant recipients and status of sarcopenia cannot be improved by LT surgery. Tsien et al. reported their finding on a prospective study of sarcopenia based on age and gender controls with a follow-up period of 19.3 months, on average (range, 11.3–28.3 months) (77). They showed that sarcopenia was common following transplantation up to 26.4% of the patient and was independent of pre-transplant characteristics such as the immunosuppressive agents, MELD score as well as Child-Pugh score. The increased proportion of 75% (15 in 20 patients) in de novo sarcopenia and 94% (31 in 33 patients) in persistent deteriorated sarcopenia after transplantation indicated that LT may not improve muscle status, which was supported by the work of Carias et al. that sarcopenia progresses and showed no sign of recovering within the first-year post LT (78).
Although scientific researchers bestowing great attention to the role of sarcopenia in NAFLD progression in general population, there is a paucity of counterpart in LT settings. Given the limited studies focusing on the issue, whether sarcopenia is a risk factor for NAFLD on LT settings remains inconclusive. It is reasonable to presume the existence of association between sarcopenia and NAFLD in LT context based on recent circumstantial evidence which need further direct evidence to prove it (79-81). Concretely, miRNA33a, a muscle nonspecific miRNA, is pivotal in the regulation of hepatic lipid metabolism. Previous studies demonstrated miRNA-33a was associated with hepatic steatosis phenotype in both preclinical model and clinical trial (82,83). Recently, clinical research demonstrated that serum miRNA-33a was associated with steatosis and inflammation in patients with recurrent or de novo NAFLD after LT (81). In addition to taking part in NAFLD progression post LT, miRNA-33a also play a role in regulating muscle function. Mechanism research revealed that miRNA-33a interfered myoblast proliferation by directly targeting insulin-like growth factor 1, cyclin D1 and follistatin via PI3K/Akt/mTOR signaling pathway (80). Given the fact that miRNA-33a involved in regulation of muscle function and NAFLD pathogenesis post LT, it is plausible to assume that miRNA-33a may function as a mediator participating in crosstalk between muscle and liver, which need further animal studies and clinical researches to verify it and explore the potential mechanisms.
Strategies to improve muscle status seem to be promising in abrogating NAFLD in LT settings. β-hydroxy-β-methyl-butyrate (HMB), metabolite of branched-chain amino acid leucine, has shown effects on muscles via multiple mechanisms including promoting myofibrillar protein synthesis, increasing proliferation and differentiation of muscle stem cells, preventing cell apoptosis and regulating protein degradation (84). Intriguingly, Sharawy et al. showed HMB possessed a property to alleviate IR and hepatic steatosis in rodent model (85), which indicated potential similar advantages on NAFLD in humans. Clinical trials aiming at improving muscle function in LT background are limited. The finished randomized controlled trial with 22 transplant patients enrolled and a follow-up period of 12 months evaluated the efficacy and safety of HMB in LT recipients (86). At the endpoint of trial, muscle strength improvement assessed by HG test only reached significant in HMB group (26.6±8.3 versus 33.7±7.6, P=0.001). And no side effect was reported in both groups regarding safety issue. There was an interesting phenomenon to note when evaluating fat mass by dual energy X-ray absorptiometry that level of increasing in fat tissue was lower in HMB group, which suggested a potential protective role of HMB on NAFLD via adipose-liver crosstalk and muscle-liver crosstalk. Furthermore, drug combination therapy is promising in anti-sarcopenia. Cellular model demonstrates the protective effects of combining HMB with R(+) lipoic acid on sarcopenia (87), which is urgent for preclinical in vivo studies to determine efficacy before employment in clinical trial.
Limitation and prospect
LT is an interesting model to study the natural history of chronic liver disease as it combines both donor graft and recipient factors. Investigations into animal studies and clinical researches afford great opportunity to study the interaction between graft and extrahepatic factors of recipients, which potentially promoting occurrence of both recurrent and de novo NAFLD. Although NAFLD have received much attention in recent years inasmuch as the increasing popularity among general population, there remains massive gaps to fill in current studies that focus on NAFLD in LT settings.
Firstly, preclinical researches into mechanism of NAFLD in LT models are still lacking. Although IR plays pivotal role in NAFLD as the extensive use of immunosuppressive agents, other mechanisms such as inflammation or oxidative stress are not fully understood regarding LT context. And whether inflammation and oxidative stress are universal in multi-organ crosstalk in LT recipients remains inconclusive. Secondly, to our knowledge, there is paucity of clinical trials directly study organ-specific drugs and their effect on NAFLD in LT recipients. Given the enthusiastic results of obeticholic acid in general population and combination regimen like HMB with R(+) lipoic acid in preclinical model, clinicians should think carefully and make further efforts to promote implementation in future medical practice. Thirdly, current management of NAFLD in LT settings is the same as of the NAFLD in non-transplant patients, which is based on the assumption that the natural history and mechanisms of NAFLD are the same in the transplanted and the non-transplant patients. However, according to the recent evidence based clinical consensus (International Liver Transplantation Society), high level of evidence is not available to support this supposition (88,89). Actually, it ignores the effects of LT surgery as well as the role of donor grafts. Fourthly, weak awareness of multidisciplinary team (MDT) cooperation exists among transplant community regarding NALFD in LT context. The status of NAFLD is complexed by the high incidence of metabolic syndrome and widely used immunosuppressant. Patients undergoing LT surgery are at risk of cardiovascular and renal events as well as frailty, thus need MDT to make joint efforts including cardiologists, nephrologists, nutritionists, metabolism experts and so on.
The aforementioned limitations in scientific research and clinical scenarios shed light on future directions in NAFLD researches under LT background. Deeper insights into mechanism research are warrant to identify the difference between transplant and non-transplant patients as well as developing organ-specific targets that pave the way for drug development. High-quality retrospective and prospective longitudinal clinical data are needed to develop management strategy for NAFLD post LT. Regarding management, it should base on deeper understanding in multi-organ crosstalk and natural history of NAFLD in LT settings to make formal guidelines. Transplant community should cooperate closely with specialists from other fields to form a powerful MDT, which maximizes benefits for graft recipients.
Conclusions
Despite the common risk factors known for all types of solid organ transplantation, the NAFLD in LT has unique mechanisms. Evidence has emerged that gut microbiota, adipose tissue and muscle status may be involved in the development of NAFLD in LT patients. Following LT, there is significant shifts in the microbiota and FXR may be a potential therapeutic target for NAFLD in LT settings. Nevertheless, the adipokines and cytokines disorder and local IR of adipose tissue may contribute to NAFLD process. BS are promising in controlling de novo and recurrent NAFLD with significant reduction in abdominal adipose tissue, despite the optimal timing is inconclusive. Furthermore, circumstantial evidence indicates that miRNA-33a may function as a mediator bridging sarcopenia and NAFLD post-LT. HMB treatment could improve muscle status in graft recipients and shows protective potential for NAFLD in LT settings.
It is important to acknowledge that the 3 factors described herein are intricately intertwined in promoting NAFLD in LT cases. Therefore, further animal studies are needed to deepen our understanding of mechanisms in multi-organ crosstalk and determine the one that provides more confidence as a new drug target to form efficient post-transplant clinical management. High quality clinical trials are warrant for making guidelines and developing management strategies on NAFLD after LT. MDT including specialists in LT and other fields should cooperate closely to maximize benefits for graft recipients.
Acknowledgments
Funding: This work was supported by National Natural Science Funds for Distinguished Young Scholar of China/International (grant No. 81625003); National Science and Technology Major Project of China (grant No. 2017ZX10203205); Science Funds for Young Scholar of China (grant No. 81702858); and Youth Program of Natural Science Foundation of Zhejiang Province (grant No. LQ17H160006).
Footnote
Reporting Checklist: The authors have completed the Narrative Review reporting checklist. Available at https://hbsn.amegroups.com/article/view/10.21037/hbsn-20-568/rc
Conflicts of Interest: All authors have completed the ICMJE uniform disclosure form (available at https://hbsn.amegroups.com/article/view/10.21037/hbsn-20-568/coif). The authors have no conflicts of interest to declare.
Ethical Statement: The authors are accountable for all aspects of the work in ensuring that questions related to the accuracy or integrity of any part of the work are appropriately investigated and resolved.
Open Access Statement: This is an Open Access article distributed in accordance with the Creative Commons Attribution-NonCommercial-NoDerivs 4.0 International License (CC BY-NC-ND 4.0), which permits the non-commercial replication and distribution of the article with the strict proviso that no changes or edits are made and the original work is properly cited (including links to both the formal publication through the relevant DOI and the license). See: https://creativecommons.org/licenses/by-nc-nd/4.0/.
References
- Dutkowski P, Linecker M, DeOliveira ML, et al. Challenges to liver transplantation and strategies to improve outcomes. Gastroenterology 2015;148:307-23. [Crossref] [PubMed]
- Schlachterman A, Craft WW Jr, Hilgenfeldt E, et al. Current and future treatments for hepatocellular carcinoma. World J Gastroenterol 2015;21:8478-91. [Crossref] [PubMed]
- Wong RJ, Cheung R, Ahmed A. Nonalcoholic steatohepatitis is the most rapidly growing indication for liver transplantation in patients with hepatocellular carcinoma in the U.S. Hepatology 2014;59:2188-95. [Crossref] [PubMed]
- Younossi ZM, Stepanova M, Ong J, et al. Nonalcoholic Steatohepatitis is the Most Rapidly Increasing Indication for Liver Transplantation in the United States. Clin Gastroenterol Hepatol 2021;19:580-9.e5. [PubMed]
- Saeed N, Glass L, Sharma P, et al. Incidence and Risks for Nonalcoholic Fatty Liver Disease and Steatohepatitis Post-liver Transplant: Systematic Review and Meta-analysis. Transplantation 2019;103:e345-e354. [Crossref] [PubMed]
- Gitto S, Marra F, De Maria N, et al. Nonalcoholic steatohepatitis before and after liver transplant: keeping up with the times. Expert Rev Gastroenterol Hepatol 2019;13:173-8. [Crossref] [PubMed]
- Gitto S, Villa E. Non-Alcoholic Fatty Liver Disease and Metabolic Syndrome after Liver Transplant. Int J Mol Sci 2016;17:490. [Crossref] [PubMed]
- Kappus M, Abdelmalek M. De Novo and Recurrence of Nonalcoholic Steatohepatitis After Liver Transplantation. Clin Liver Dis 2017;21:321-35. [Crossref] [PubMed]
- Barchetta I, Angelico F, Del Ben M, et al. Strong association between non alcoholic fatty liver disease (NAFLD) and low 25(OH) vitamin D levels in an adult population with normal serum liver enzymes. BMC Med 2011;9:85. [Crossref] [PubMed]
- Chiu KW, Goto S, Nakano T, et al. Genetic polymorphisms of the hepatic pathways of fatty liver disease after living donor liver transplantation. Liver Int 2018;38:2287-93. [Crossref] [PubMed]
- Li JZ, Huang Y, Karaman R, et al. Chronic overexpression of PNPLA3I148M in mouse liver causes hepatic steatosis. J Clin Invest 2012;122:4130-44. [Crossref] [PubMed]
- Trunečka P, Mikova I, Dlouha D, et al. Donor PNPLA3 rs738409 genotype is a risk factor for graft steatosis. A post-transplant biopsy-based study. Dig Liver Dis 2018;50:490-5. [Crossref] [PubMed]
- Hong HC, Hwang SY, Choi HY, et al. Relationship between sarcopenia and nonalcoholic fatty liver disease: the Korean Sarcopenic Obesity Study. Hepatology 2014;59:1772-8. [Crossref] [PubMed]
- Miyaaki H, Miuma S, Taura N, et al. Risk Factors and Clinical Course for Liver Steatosis or Nonalcoholic Steatohepatitis After Living Donor Liver Transplantation. Transplantation 2019;103:109-12. [Crossref] [PubMed]
- Yu Y, Cai J, She Z, et al. Insights into the Epidemiology, Pathogenesis, and Therapeutics of Nonalcoholic Fatty Liver Diseases. Adv Sci (Weinh) 2018;6:1801585. [Crossref] [PubMed]
- Bajaj JS, Kakiyama G, Cox IJ, et al. Alterations in gut microbial function following liver transplant. Liver Transpl 2018;24:752-61. [Crossref] [PubMed]
- Wu ZW, Ling ZX, Lu HF, et al. Changes of gut bacteria and immune parameters in liver transplant recipients. Hepatobiliary Pancreat Dis Int 2012;11:40-50. [Crossref] [PubMed]
- Mouries J, Brescia P, Silvestri A, et al. Microbiota-driven gut vascular barrier disruption is a prerequisite for non-alcoholic steatohepatitis development. J Hepatol 2019;71:1216-28. [Crossref] [PubMed]
- Zhu L, Baker SS, Gill C, et al. Characterization of gut microbiomes in nonalcoholic steatohepatitis (NASH) patients: a connection between endogenous alcohol and NASH. Hepatology 2013;57:601-9. [Crossref] [PubMed]
- Bajaj JS, Fagan A, Sikaroodi M, et al. Liver transplant modulates gut microbial dysbiosis and cognitive function in cirrhosis. Liver Transpl 2017;23:907-14. [Crossref] [PubMed]
- Xu RY, Wan YP, Fang QY, et al. Supplementation with probiotics modifies gut flora and attenuates liver fat accumulation in rat nonalcoholic fatty liver disease model. J Clin Biochem Nutr 2012;50:72-7. [Crossref] [PubMed]
- Berná G, Romero-Gomez M. The role of nutrition in non-alcoholic fatty liver disease: Pathophysiology and management. Liver Int 2020;40:102-8. [Crossref] [PubMed]
- Abenavoli L, Boccuto L, Federico A, et al. Diet and Non-Alcoholic Fatty Liver Disease: The Mediterranean Way. Int J Environ Res Public Health 2019;16:3011. [Crossref] [PubMed]
- Krawczyk M, Maciejewska D, Ryterska K, et al. Gut Permeability Might be Improved by Dietary Fiber in Individuals with Nonalcoholic Fatty Liver Disease (NAFLD) Undergoing Weight Reduction. Nutrients 2018;10:1793. [Crossref] [PubMed]
- Kobyliak N, Abenavoli L, Mykhalchyshyn G, et al. A Multi-strain Probiotic Reduces the Fatty Liver Index, Cytokines and Aminotransferase levels in NAFLD Patients: Evidence from a Randomized Clinical Trial. J Gastrointestin Liver Dis 2018;27:41-9. [Crossref] [PubMed]
- Abenavoli L, Di Renzo L, Boccuto L, et al. Health benefits of Mediterranean diet in nonalcoholic fatty liver disease. Expert Rev Gastroenterol Hepatol 2018;12:873-81. [Crossref] [PubMed]
- Wong VW, Won GL, Chim AM, et al. Treatment of nonalcoholic steatohepatitis with probiotics. A proof-of-concept study. Ann Hepatol 2013;12:256-62. [Crossref] [PubMed]
- Cheng L, Tian F, Tian F, et al. Repression of Farnesoid X receptor contributes to biliary injuries of liver grafts through disturbing cholangiocyte bile acid transport. Am J Transplant 2013;13:3094-102. [Crossref] [PubMed]
- Neuschwander-Tetri BA, Loomba R, Sanyal AJ, et al. Farnesoid X nuclear receptor ligand obeticholic acid for non-cirrhotic, non-alcoholic steatohepatitis (FLINT): a multicentre, randomised, placebo-controlled trial. Lancet 2015;385:956-65. [Crossref] [PubMed]
- Fujino T, Murakami K, Ozawa I, et al. Hypoxia downregulates farnesoid X receptor via a hypoxia-inducible factor-independent but p38 mitogen-activated protein kinase-dependent pathway. FEBS J 2009;276:1319-32. [Crossref] [PubMed]
- Chávez-Talavera O, Tailleux A, Lefebvre P, et al. Bile Acid Control of Metabolism and Inflammation in Obesity, Type 2 Diabetes, Dyslipidemia, and Nonalcoholic Fatty Liver Disease. Gastroenterology 2017;152:1679-94.e3. [Crossref] [PubMed]
- Younossi ZM, Ratziu V, Loomba R, et al. Obeticholic acid for the treatment of non-alcoholic steatohepatitis: interim analysis from a multicentre, randomised, placebo-controlled phase 3 trial. Lancet 2019;394:2184-96. [Crossref] [PubMed]
- Anastácio LR, Pereira MC, Vilela EG, et al. Overweight in liver transplant recipients. Rev Col Bras Cir 2013;40:502-7. [PubMed]
- Said A. Non-alcoholic fatty liver disease and liver transplantation: outcomes and advances. World J Gastroenterol 2013;19:9146-55. [Crossref] [PubMed]
- Lattanzi B, D'Ambrosio D, Tavano D, et al. Weight Gain and De Novo Metabolic Disorders after Liver Transplantation. Nutrients 2019;11:3015. [Crossref] [PubMed]
- Bijnen M, Josefs T, Cuijpers I, et al. Adipose tissue macrophages induce hepatic neutrophil recruitment and macrophage accumulation in mice. Gut 2018;67:1317-27. [Crossref] [PubMed]
- Tejedor-Tejada J, Valenzuela EF, Munoz RN, et al. De-novo nonalcoholic fatty liver disease at 5 years after liver transplantation: prevalence and predictive factors. Eur J Gastroenterol Hepatol 2021;33:399-406. [PubMed]
- Kershaw EE, Flier JS. Adipose tissue as an endocrine organ. J Clin Endocrinol Metab 2004;89:2548-56. [Crossref] [PubMed]
- La Cava A. Leptin in inflammation and autoimmunity. Cytokine 2017;98:51-8. [Crossref] [PubMed]
- Imajo K, Fujita K, Yoneda M, et al. Hyperresponsivity to low-dose endotoxin during progression to nonalcoholic steatohepatitis is regulated by leptin-mediated signaling. Cell Metab 2012;16:44-54. [Crossref] [PubMed]
- Roberts GA, Holt RI, Ghatei MA, et al. Serum leptin and insulin in paediatric end-stage liver disease and following successful orthotopic liver transplantation. Clin Endocrinol (Oxf) 1998;48:401-6. [Crossref] [PubMed]
- Watt KD, Fan C, Therneau T, et al. Serum adipokine and inflammatory markers before and after liver transplantation in recipients with major cardiovascular events. Liver Transpl 2014;20:791-7. [Crossref] [PubMed]
- Polyzos SA, Aronis KN, Kountouras J, et al. Circulating leptin in non-alcoholic fatty liver disease: a systematic review and meta-analysis. Diabetologia 2016;59:30-43. [Crossref] [PubMed]
- Casey SP, Lokan J, Testro A, et al. Post-liver transplant leptin results in resolution of severe recurrence of lipodystrophy-associated nonalcoholic steatohepatitis. Am J Transplant 2013;13:3031-4. [Crossref] [PubMed]
- Abenavoli L, Peta V. Role of adipokines and cytokines in non-alcoholic fatty liver disease. Rev Recent Clin Trials 2014;9:134-40. [Crossref] [PubMed]
- Kumar R, Prakash S, Chhabra S, et al. Association of pro-inflammatory cytokines, adipokines & oxidative stress with insulin resistance & non-alcoholic fatty liver disease. Indian J Med Res 2012;136:229-36. [PubMed]
- Hui JM, Hodge A, Farrell GC, et al. Beyond insulin resistance in NASH: TNF-alpha or adiponectin? Hepatology 2004;40:46-54. [Crossref] [PubMed]
- Nov O, Shapiro H, Ovadia H, et al. Interleukin-1beta regulates fat-liver crosstalk in obesity by auto-paracrine modulation of adipose tissue inflammation and expandability. PLoS One 2013;8:e53626. [Crossref] [PubMed]
- Hart KM, Fabre T, Sciurba JC, et al. Type 2 immunity is protective in metabolic disease but exacerbates NAFLD collaboratively with TGF-β. Sci Transl Med 2017;9:eaal3694. [Crossref] [PubMed]
- Alvares-da-Silva MR, de Oliveira CP, Stefano JT, et al. Pro-atherosclerotic markers and cardiovascular risk factors one year after liver transplantation. World J Gastroenterol 2014;20:8667-73. [Crossref] [PubMed]
- Watt KD, Pedersen RA, Kremers WK, et al. Evolution of causes and risk factors for mortality post-liver transplant: results of the NIDDK long-term follow-up study. Am J Transplant 2010;10:1420-7. [Crossref] [PubMed]
- Pereira MJ, Palming J, Rizell M, et al. Cyclosporine A and tacrolimus reduce the amount of GLUT4 at the cell surface in human adipocytes: increased endocytosis as a potential mechanism for the diabetogenic effects of immunosuppressive agents. J Clin Endocrinol Metab 2014;99:E1885-94. [Crossref] [PubMed]
- Fonseca AC, Carvalho E, Eriksson JW, et al. Calcineurin is an important factor involved in glucose uptake in human adipocytes. Mol Cell Biochem 2018;445:157-68. [Crossref] [PubMed]
- Rondinone CM, Smith U. Okadaic acid exerts a full insulin-like effect on glucose transport and glucose transporter 4 translocation in human adipocytes. Evidence for a phosphatidylinositol 3-kinase-independent pathway. J Biol Chem 1996;271:18148-53. [Crossref] [PubMed]
- Pereira MJ, Palming J, Rizell M, et al. mTOR inhibition with rapamycin causes impaired insulin signalling and glucose uptake in human subcutaneous and omental adipocytes. Mol Cell Endocrinol 2012;355:96-105. [Crossref] [PubMed]
- Chakkera HA, Kudva Y, Kaplan B. Calcineurin Inhibitors: Pharmacologic Mechanisms Impacting Both Insulin Resistance and Insulin Secretion Leading to Glucose Dysregulation and Diabetes Mellitus. Clin Pharmacol Ther 2017;101:114-20. [Crossref] [PubMed]
- Hazlehurst JM, Gathercole LL, Nasiri M, et al. Glucocorticoids fail to cause insulin resistance in human subcutaneous adipose tissue in vivo. J Clin Endocrinol Metab 2013;98:1631-40. [Crossref] [PubMed]
- Sidibeh CO, Pereira MJ, Lau Borjesson J, et al. Role of cannabinoid receptor 1 in human adipose tissue for lipolysis regulation and insulin resistance. Endocrine 2017;55:839-52. [Crossref] [PubMed]
- Sidibeh CO, Pereira MJ, Abalo XM, et al. FKBP5 expression in human adipose tissue: potential role in glucose and lipid metabolism, adipogenesis and type 2 diabetes. Endocrine 2018;62:116-28. [Crossref] [PubMed]
- Balsevich G, Hausl AS, Meyer CW, et al. Stress-responsive FKBP51 regulates AKT2-AS160 signaling and metabolic function. Nat Commun 2017;8:1725. [Crossref] [PubMed]
- Wipperman MF, Montrose DC, Gotto AM Jr, et al. Mammalian Target of Rapamycin: A Metabolic Rheostat for Regulating Adipose Tissue Function and Cardiovascular Health. Am J Pathol 2019;189:492-501. [Crossref] [PubMed]
- Chaveroux C, Eichner LJ, Dufour CR, et al. Molecular and genetic crosstalks between mTOR and ERRalpha are key determinants of rapamycin-induced nonalcoholic fatty liver. Cell Metab 2013;17:586-98. [Crossref] [PubMed]
- Kwak M, Mehaffey JH, Hawkins RB, et al. Bariatric surgery is associated with reduction in non-alcoholic steatohepatitis and hepatocellular carcinoma: A propensity matched analysis. Am J Surg 2020;219:504-7. [Crossref] [PubMed]
- Buchwald H, Estok R, Fahrbach K, et al. Weight and type 2 diabetes after bariatric surgery: systematic review and meta-analysis. Am J Med 2009;122:248-56.e5. [Crossref] [PubMed]
- Diwan TS, Rice TC, Heimbach JK, et al. Liver Transplantation and Bariatric Surgery: Timing and Outcomes. Liver Transpl 2018;24:1280-7. [Crossref] [PubMed]
- Idriss R, Hasse J, Wu T, et al. Impact of Prior Bariatric Surgery on Perioperative Liver Transplant Outcomes. Liver Transpl 2019;25:217-27. [Crossref] [PubMed]
- Ayloo S, Guss C, Pentakota SR, et al. Minimally Invasive Sleeve Gastrectomy as a Surgical Treatment for Nonalcoholic Fatty Liver Disease in Liver Transplant Recipients. Transplant Proc 2020;52:276-83. [Crossref] [PubMed]
- Golse N, Bucur PO, Ciacio O, et al. A new definition of sarcopenia in patients with cirrhosis undergoing liver transplantation. Liver Transpl 2017;23:143-54. [Crossref] [PubMed]
- Masuda T, Shirabe K, Ikegami T, et al. Sarcopenia is a prognostic factor in living donor liver transplantation. Liver Transpl 2014;20:401-7. [Crossref] [PubMed]
- Durand F, Buyse S, Francoz C, et al. Prognostic value of muscle atrophy in cirrhosis using psoas muscle thickness on computed tomography. J Hepatol 2014;60:1151-7. [Crossref] [PubMed]
- Carey EJ, Lai JC, Sonnenday C, et al. A North American Expert Opinion Statement on Sarcopenia in Liver Transplantation. Hepatology 2019;70:1816-29. [Crossref] [PubMed]
- Lee YH, Kim SU. Sarcopenia: an emerging risk factor for non-alcoholic fatty liver disease. Hepatol Int 2020;14:5-7. [Crossref] [PubMed]
- El Sherif O, Dhaliwal A, Newsome PN, et al. Sarcopenia in nonalcoholic fatty liver disease: new challenges for clinical practice. Expert Rev Gastroenterol Hepatol 2020;14:197-205. [Crossref] [PubMed]
- Koo BK, Kim D, Joo SK, et al. Sarcopenia is an independent risk factor for non-alcoholic steatohepatitis and significant fibrosis. J Hepatol 2017;66:123-31. [Crossref] [PubMed]
- Cai C, Song X, Chen Y, et al. Relationship between relative skeletal muscle mass and nonalcoholic fatty liver disease: a systematic review and meta-analysis. Hepatol Int 2020;14:115-26. [Crossref] [PubMed]
- Merli M, Dasarathy S. Sarcopenia in non-alcoholic fatty liver disease: Targeting the real culprit? J Hepatol 2015;63:309-11. [Crossref] [PubMed]
- Tsien C, Garber A, Narayanan A, et al. Post-liver transplantation sarcopenia in cirrhosis: a prospective evaluation. J Gastroenterol Hepatol 2014;29:1250-7. [Crossref] [PubMed]
- Carias S, Castellanos AL, Vilchez V, et al. Nonalcoholic steatohepatitis is strongly associated with sarcopenic obesity in patients with cirrhosis undergoing liver transplant evaluation. J Gastroenterol Hepatol 2016;31:628-33. [Crossref] [PubMed]
- Zhang C, Chen K, Wei R, et al. The circFASN/miR-33a pathway participates in tacrolimus-induced dysregulation of hepatic triglyceride homeostasis. Signal Transduct Target Ther 2020;5:23. [Crossref] [PubMed]
- Li X, Qiu J, Liu H, et al. MicroRNA-33a negatively regulates myoblast proliferation by targeting IGF1, follistatin and cyclin D1. Biosci Rep 2020;40:BSR20191327. [Crossref] [PubMed]
- Erhartova D, Cahova M, Dankova H, et al. Serum miR-33a is associated with steatosis and inflammation in patients with non-alcoholic fatty liver disease after liver transplantation. PLoS One 2019;14:e0224820. [Crossref] [PubMed]
- Vega-Badillo J, Gutierrez-Vidal R, Hernandez-Perez HA, et al. Hepatic miR-33a/miR-144 and their target gene ABCA1 are associated with steatohepatitis in morbidly obese subjects. Liver Int 2016;36:1383-91. [Crossref] [PubMed]
- Horie T, Nishino T, Baba O, et al. MicroRNA-33 regulates sterol regulatory element-binding protein 1 expression in mice. Nat Commun 2013;4:2883. [Crossref] [PubMed]
- Cruz-Jentoft AJ. Beta-Hydroxy-Beta-Methyl Butyrate (HMB): From Experimental Data to Clinical Evidence in Sarcopenia. Curr Protein Pept Sci 2018;19:668-72. [Crossref] [PubMed]
- Sharawy MH, El-Awady MS, Megahed N, et al. The ergogenic supplement beta-hydroxy-beta-methylbutyrate (HMB) attenuates insulin resistance through suppressing GLUT-2 in rat liver. Can J Physiol Pharmacol 2016;94:488-97. [Crossref] [PubMed]
- Lattanzi B, Giusto M, Albanese C, et al. The Effect of 12 Weeks of β-Hydroxy-β-Methyl-Butyrate Supplementation after Liver Transplantation: A Pilot Randomized Controlled Study. Nutrients 2019;11:2259. [Crossref] [PubMed]
- Di Cesare Mannelli L, Micheli L, Lucarini E, et al. Effects of the Combination of β-Hydroxy-β-Methyl Butyrate and R(+) Lipoic Acid in a Cellular Model of Sarcopenia. Molecules 2020;25:2117. [Crossref] [PubMed]
- Germani G, Laryea M, Rubbia-Brandt L, et al. Management of Recurrent and De Novo NAFLD/NASH After Liver Transplantation. Transplantation 2019;103:57-67. [Crossref] [PubMed]
- Burra P, Berenguer M, Pomfret E. The ILTS Consensus Conference on NAFLD/NASH and Liver Transplantation: Setting the Stage. Transplantation 2019;103:19-21. [Crossref] [PubMed]