Clinical application of indocyanine green-fluorescence imaging during hepatectomy
Overview of intraoperative fluorescence imaging using indocyanine green (ICG)
Since approval by the U.S. Food and Drug Administration (FDA) in 1954, ICG has been used clinically to estimate cardiac output and liver function. In the 1970s, protein-bound ICG was found to emit fluorescence, peaking at about 840 nm, under illumination with near-infrared light (750−810 nm) (1). Because little light at 840 nm is absorbed by hemoglobin or water, fluorescence signals emitted by protein-bound ICG can be visualized through connective tissue 5−10 mm thick. ICG was first used clinically for fundus angiography in the early 1990s (2).
In the 21st century, fluorescence imaging using ICG has become widespread as an intraoperative navigation tool to detect lymphatic flow in the extremities (3); sentinel lymph nodes in patients with breast (4) and gastric (5) cancers; and blood flow during coronary artery bypass grafting (6) and clipping of cerebral artery aneurysms (7). In hepatobiliary surgery, however, little attention was paid to the fluorescence of ICG until, in the late 2000s, Japanese surgeons used ICG-fluorescence imaging to visualize hepatobiliary structures (8-11). This is probably because liver surgeons regarded ICG as a reagent for estimation of hepatic function.
Potentially, ICG-fluorescence imaging is highly suitable for hepatobiliary surgery, because the fluorescence of ICG and its biliary excretion can be used for the intraoperative identification of biological structures. In 2009, the first report of fluorescence cholangiography during laparoscopic cholecystectomy described using ICG excreted into bile following preoperative intravenous injection as the source of fluorescence (12). During the development of fluorescence cholangiography for hepatic tumors, it was found that ICG accumulated in cancerous tissues of hepatocellular carcinoma (HCC) and in non-cancerous hepatic parenchyma around adenocarcinoma foci (13,14). Recently, this technique has been used clinically to identify hepatic tumors during laparoscopic hepatectomy (15), and during open surgery. Refinements in imaging techniques have enabled the use of ICG-fluorescence imaging for visualization of hepatic segments, enabling more accurate anatomic resection of the liver (16,17).
Fluorescence cholangiography
Because human bile contains proteins such as albumin and lipoproteins that bind ICG (18), fluorescent images of the biliary tract can be obtained by intrabiliary injection of ICG (11). The fluorescence intensity of protein-bound ICG was found to correlate with its concentrations to approximately 0.25 mg/mL, decreasing at higher concentrations because of the absorption of near-infrared light by ICG (9). Thus, to obtain clear fluorescence images of the bile ducts following intrabiliary injection of ICG, diluted ICG solution (approximately 0.025 mg/mL) should be used for imaging (11). It is also important to aspirate a small amount of bile into the syringe before injection to promote binding of ICG to proteins. When the intrahepatic bile duct anatomy and the extrahepatic biliary system must be identified, ICG should be diluted with radiographic contrast agents, enabling radiographic cholangiography easily and immediately following fluorescence cholangiography (19).
Fluorescence cholangiography could also be performed following intravenous injection of ICG, because ICG excreted into bile can act as a source of fluorescence (Figure 1) (7). This technique involves the intravenous injection of small amounts of ICG, usually 2.5 mg, diluted into 1 mL solution (12,20). Although biliary excretion of ICG begins within minutes after intravenous injection (21), ICG should be administered at least 15 minutes before imaging to obtain better signal-to-background contrast. Indeed, ICG fluorescence in the extrahepatic bile ducts continues up to 6 hours after injection (12). Intravenous injection of ICG has potential advantages over conventional radiographic cholangiography in saving time and avoiding bile duct injury associated with the catheterization required for injection of contrast materials. Although fluorescence cholangiography has a limitation in detecting small stones floating in the common bile duct, the present technique has recently gained attention as a novel and easy-to-use navigation tool that provides a roadmap of the extrahepatic ducts, enhancing safety (22) during laparoscopic (23-28) and robotic (29,30) cholecystectomy and reducing the need for intraoperative radiographic cholangiography.
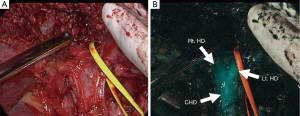
Fluorescence imaging of hepatic tumors
In a previous study of 37 patients with HCC and 12 with colorectal liver metastasis (CRLM), fluorescence imaging following preoperative intravenous injection of 0.5 mg/kg ICG identified all of the microscopically confirmed HCCs and CRLM on the cut surfaces of the resected specimens (13). The fluorescence patterns of these tumors could be classified into three types: total fluorescence, in which all tumor tissue showed uniform fluorescence; partial fluorescence, in which some tumor tissues showed fluorescence; and rim fluorescence, in which the cancer tissues were negative for fluorescence, but the surrounding liver parenchyma showed fluorescence. These fluorescence patterns were closely associated with the characteristics of the liver cancers. Total fluorescence-type tumors included all well-differentiated HCCs, whereas rim fluorescence-type-tumors consisted only of poorly differentiated HCCs and CRLM.
Recently, the mechanism of ICG-fluorescence imaging of HCCs was elucidated by immunohistochemical staining and gene expression analysis (14). In differentiated HCC tissues, the expression levels of portal uptake transporters of ICG [organic anion-transporting polypeptide 8 and Na+/taurocholate cotransporting polypeptide (31)] were well preserved, but functional or morphological biliary excretion disorders were present, leading to retention of ICG in cancerous tissues at the time of surgery, following preoperative intravenous injection. In poorly-differentiated HCCs, however, the portal uptake transporters were downregulated in cancerous tissues but biliary excretion of ICG by surrounding non-cancerous hepatic parenchyma was also disordered, resulting in rim-type fluorescence. The rim-type fluorescence signal in CRLM has been reported to be caused by immature hepatocytes with decreased bile excretion ability that surrounds the tumor (32). ICG fluorescence of HCC tissues was found associated with a risk of recurrence after hepatectomy (33).
Irrespective of their fluorescence patterns, subcapsular hepatic tumors can be identified on the liver surfaces by intraoperative fluorescence imaging, following preoperative intravenous injection of ICG. In this technique, ICG (0.5 mg/kg body weight) is administered intravenously, usually within two weeks before surgery. This method can also be used to detect biliary congestion caused by tumor invasion (34), micrometastases from pancreatic cancer (35), and extrahepatic spread of HCC (36). The intraoperative ICG-fluorescence imaging of hepatic tumors is simple and is especially useful for identifying subcapsular lesions for removal during laparoscopic hepatectomy, in which visual inspection and palpation are limited compared with open surgery (Figure 2) (15).
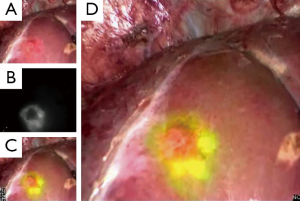
This technique has potential drawbacks, however, including a relatively high false-positive rate [around 40% (13,14)]. Lesions newly detected by ICG-fluorescence imaging should be resected only when other modalities, such as palpation and intraoperative ultrasonography, identify them as tumors to be removed. The incidence of false-positives can be reduced by not administering ICG on the day before surgery, especially in patients with decreased liver function due to cirrhosis or preoperative chemotherapy (13).
Fluorescence imaging of hepatic segments
Anatomic segmentectomy is the essential surgical technique in hepatectomy, balancing cancer curability and postoperative hepatic function (37). Boundaries of hepatic segments prior to anatomic resection can be identified by a dye-staining technique, in which indigo-carmine solution is injected into the corresponding portal branch under ultrasound guidance, with positivity defined as blue staining of hepatic surfaces. In 2008, fluorescence imaging following portal injection of ICG was first used in the intraoperative identification of hepatic segments (10). This technique was later refined (16) by using a more diluted solution of ICG (2.5 mg) as a source of fluorescence and an imaging system enabling fusion of fluorescence images on color images. The concomitant injection of indigo-carmine solution with a small amount of ICG (0.25 mg) could enhance the success rate of hepatic segment identification, especially in patients with cirrhosis and/or those having livers covered by thick connective tissues owing to previous surgery (17) (Figure 3).
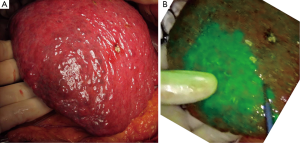
In addition to identifying hepatic segments for removal following portal injection of ICG solution (positive staining technique), hepatic segments can be identified as ischemic regions by intravenous injection of ICG (2.5 mg) following closure/division of the corresponding portal pedicle (negative staining technique) (16,38). The latter technique is especially useful in laparoscopic hepatic segmentectomy, in which injection of ICG solution into the portal vein is technically difficult (38,39). Intraoperative fluorescence imaging can also be used to estimate portal uptake function in veno-occlusive regions of the liver during hepatectomy or living-donor liver transplantation, by measuring trends of fluorescence intensity of the hepatic surfaces following injection of ICG (40,41).
Future prospects of ICG-fluorescence imaging in hepatobiliary surgery
In laparoscopic and robotic surgery, surgical procedures are based on operation fields displayed on a monitor. Thus, it is not surprising that fluorescence imaging was rapidly applied to these minimally-invasive methods. Laparoscopic and robotic fluorescence imaging systems have become commercially available (Figure 4A). In the near future, three-dimensional and ultra-high definition imaging (4K) may be delivered to clinical settings. Further technological innovations, however, are need to improve the feasibility of fluorescence imaging during open surgery, enabling surgeons to operate without having to switch from operation fields to a TV monitor (Figure 4B).
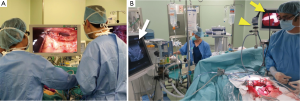
Acknowledgements
The authors would like to thank Dr. Yoshihiro Mise, Dr. Yosuke Inoue, and Dr. Yu Takahashi for their contributions to applying fluorescence imaging to hepatobiliary and pancreatic surgeries.
Funding: This work was supported by grants from the Mochida Memorial Foundation for Medical and Pharmaceutical Research; the Ministry of Education, Culture, Sports, Science and Technology of Japan (No. 23689060); and the Ministry of Health, Labour and Welfare of Japan.
Footnote
Conflicts of Interest: The authors have no conflicts of interest to declare.
References
- Landsman ML, Kwant G, Mook GA, et al. Light-absorbing properties, stability, and spectral stabilization of indocyanine green. J Appl Physiol 1976;40:575-83. [PubMed]
- Guyer DR, Puliafito CA, Monés JM, et al. Digital indocyanine-green angiography in chorioretinal disorders. Ophthalmology 1992;99:287-91. [Crossref] [PubMed]
- Ogata F, Azuma R, Kikuchi M, et al. Novel lymphography using indocyanine green dye for near-infrared fluorescence labeling. Ann Plast Surg 2007;58:652-5. [Crossref] [PubMed]
- Kitai T, Inomoto T, Miwa M, et al. Fluorescence navigation with indocyanine green for detecting sentinel lymph nodes in breast cancer. Breast Cancer 2005;12:211-5. [Crossref] [PubMed]
- Kusano M, Tajima Y, Yamazaki K, et al. Sentinel node mapping guided by indocyanine green fluorescence imaging: a new method for sentinel node navigation surgery in gastrointestinal cancer. Dig Surg 2008;25:103-8. [Crossref] [PubMed]
- Rubens FD, Ruel M, Fremes SE. A new and simplified method for coronary and graft imaging during CABG. Heart Surg Forum 2002;5:141-4. [PubMed]
- Raabe A, Nakaji P, Beck J, et al. Prospective evaluation of surgical microscope-integrated intraoperative near-infrared indocyanine green videoangiography during aneurysm surgery. J Neurosurg 2005;103:982-9. [Crossref] [PubMed]
- Kubota K, Kita J, Shimoda M, et al. Intraoperative assessment of reconstructed vessels in living-donor liver transplantation, using a novel fluorescence imaging technique. J Hepatobiliary Pancreat Surg 2006;13:100-4. [Crossref] [PubMed]
- Mitsuhashi N, Kimura F, Shimizu H, et al. Usefulness of intraoperative fluorescence imaging to evaluate local anatomy in hepatobiliary surgery. J Hepatobiliary Pancreat Surg 2008;15:508-14. [Crossref] [PubMed]
- Aoki T, Yasuda D, Shimizu Y, et al. Image-guided liver mapping using fluorescence navigation system with indocyanine green for anatomical hepatic resection. World J Surg 2008;32:1763-7. [Crossref] [PubMed]
- Ishizawa T, Tamura S, Masuda K, et al. Intraoperative fluorescent cholangiography using indocyanine green: a biliary road map for safe surgery. J Am Coll Surg 2009;208:e1-4. [Crossref] [PubMed]
- Ishizawa T, Bandai Y, Kokudo N. Fluorescent cholangiography using indocyanine green for laparoscopic cholecystectomy: an initial experience. Arch Surg 2009;144:381-2. [Crossref] [PubMed]
- Ishizawa T, Fukushima N, Shibahara J, et al. Real-time identification of liver cancers by using indocyanine green fluorescent imaging. Cancer 2009;115:2491-504. [Crossref] [PubMed]
- Ishizawa T, Masuda K, Urano Y, et al. Mechanistic background and clinical applications of indocyanine green fluorescence imaging of hepatocellular carcinoma. Ann Surg Oncol 2014;21:440-8. [Crossref] [PubMed]
- Kudo H, Ishizawa T, Tani K, et al. Visualization of subcapsular hepatic malignancy by indocyanine-green fluorescence imaging during laparoscopic hepatectomy. Surg Endosc 2014;28:2504-8. [Crossref] [PubMed]
- Inoue Y, Arita J, Sakamoto T, et al. Anatomical liver resections guided by 3-dimensional parenchymal staining using fusion indocyanine green fluorescence imaging. Ann Surg 2015;262:105-11. [Crossref] [PubMed]
- Miyata A, Ishizawa T, Tani K, et al. Reappraisal of a dye-staining technique for anatomic hepatectomy by the concomitant use of indocyanine green fluorescence imaging. J Am Coll Surg 2015;221:e27-36. [Crossref] [PubMed]
- Mullock BM, Shaw LJ, Fitzharris B, et al. Sources of proteins in human bile. Gut 1985;26:500-9. [Crossref] [PubMed]
- Ishizawa T, Kokudo N. Fluorescence imaging of human bile and biliary anatomy. In: Fong Y, Giulianotti PC, Lewis J, et al, editors. Imaging and visualization in the modern operating room. New York: Springer, 2015:271-8.
- Ishizawa T, Bandai Y, Ijichi M, et al. Fluorescent cholangiography illuminating the biliary tree during laparoscopic cholecystectomy. Br J Surg 2010;97:1369-77. [Crossref] [PubMed]
- Cherrick GR, Stein SW, Leevy CM, et al. Indocyanine green: observations on its physical properties, plasma decay, and hepatic extraction. J Clin Invest 1960;39:592-600. [Crossref] [PubMed]
- Strasberg SM, Hertl M, Soper NJ. An analysis of the problem of biliary injury during laparoscopic cholecystectomy. J Am Coll Surg 1995;180:101-25. [PubMed]
- Tagaya N, Shimoda M, Kato M, et al. Intraoperative exploration of biliary anatomy using fluorescence imaging of indocyanine green in experimental and clinical cholecystectomies. J Hepatobiliary Pancreat Sci 2010;17:595-600. [Crossref] [PubMed]
- Sherwinter DA. Identification of anomolous biliary anatomy using near-infrared cholangiography. J Gastrointest Surg 2012;16:1814-5. [Crossref] [PubMed]
- Osayi SN, Wendling MR, Drosdeck JM, et al. Near-infrared fluorescent cholangiography facilitates identification of biliary anatomy during laparoscopic cholecystectomy. Surg Endosc 2015;29:368-75. [Crossref] [PubMed]
- Schols RM, Bouvy ND, van Dam RM, et al. Combined vascular and biliary fluorescence imaging in laparoscopic cholecystectomy. Surg Endosc 2013;27:4511-7. [Crossref] [PubMed]
- Verbeek FP, Schaafsma BE, Tummer QR, et al. Optimization of near-infrared fluorescence cholangiography for open and laparoscopic surgery. Surg Endosc 2014;28:1076-82. [Crossref] [PubMed]
- Dip F, Roy M, Lo Menzo E, et al. Routine use of fluorescent incisionless cholangiography as a new imaging modality during laparoscopic cholecystectomy. Surg Endosc 2015;29:1621-6. [Crossref] [PubMed]
- Spinoglio G, Priora F, Bianchi PP, et al. Real-time near-infrared (NIR) fluorescent cholangiography in single-site robotic cholecystectomy (SSRC): a single-institutional prospective study. Surg Endosc 2013;27:2156-62. [Crossref] [PubMed]
- Daskalaki D, Fernandes E, Wang X, et al. Indocyanine green (ICG) fluorescent cholangiography during robotic cholecystectomy: results of 184 consecutive cases in a single institution. Surg Innov 2014;21:615-21. [Crossref] [PubMed]
- de Graaf W, Häusler S, Heger M, et al. Transporters involved in the hepatic uptake of (99m)Tc-mebrofenin and indocyanine green. J Hepatol 2011;54:738-45. [Crossref] [PubMed]
- van der Vorst JR, Schaafsma BE, Hutteman M, et al. Near-infrared fluorescence-guided resection of colorectal liver metastases. Cancer 2013;119:3411-8. [Crossref] [PubMed]
- Shibasaki Y, Sakaguchi T, Hiraide T, et al. Expression of indocyanine green-related transporters in hepatocellular carcinoma. J Surg Res 2015;193:567-76. [Crossref] [PubMed]
- Harada N, Ishizawa T, Muraoka A, et al. Fluorescence navigation hepatectomy by visualization of localized cholestasis from bile duct tumor infiltration. J Am Coll Surg 2010;210:e2-6. [Crossref] [PubMed]
- Yokoyama N, Otani T, Hashidate H, et al. Real-time detection of hepatic micrometastases from pancreatic cancer by intraoperative fluorescence imaging: Preliminary results of a prospective study. Cancer 2012;118:2813-9. [Crossref] [PubMed]
- Satou S, Ishizawa T, Kokudo N, et al. Indocyanine green fluorescent imaging for detecting extrahepatic metastasis of hepatocellular carcinoma. J Gastroenterol 2013;48:1136-43. [Crossref] [PubMed]
- Makuuchi M, Hasegawa H, Yamazaki S. Ultrasonically guided subsegmentectomy. Surg Gynecol Obstet 1985;161:346-50. [PubMed]
- Ishizawa T, Zuker NB, Kokudo N, et al. Positive and negative staining of hepatic segments by use of fluorescent imaging techniques during laparoscopic hepatectomy. Arch Surg 2012;147:393-4. [Crossref] [PubMed]
- Ishizawa T, Gumbs AA, Kokudo N, et al. Laparoscopic segmentectomy of the liver: from segment I to VIII. Ann Surg 2012;256:959-64. [Crossref] [PubMed]
- Kawaguchi Y, Ishizawa T, Miyata Y, et al. Portal uptake function in veno-occlusive regions evaluated by real-time fluorescent imaging using indocyanine green. J Hepatol 2013;58:247-53. [Crossref] [PubMed]
- Inoue Y, Saiura A, Arita J, et al. Hepatic Vein-Oriented Liver Resection Using Fusion Indocyanine Green Fluorescence Imaging. Ann Surg 2015;262:e98-9. [Crossref] [PubMed]