Large, multifocal or portal vein-invading hepatocellular carcinoma (HCC) downstaged by Y90 using personalized dosimetry: safety, pathological results and outcomes after surgery
Introduction
Y90 transarterial radioembolization (TARE) was recently endorsed in Barcelona clinic liver cancer (BCLC) recommendations (1), based on the results of the LEGACY study, which reported outcomes following TARE of ≤8 cm solitary hepatocellular carcinoma (HCC) comparable to those following curative-intent therapies (2). TARE was also supported by the high rate of complete pathological response in this population (3-5). On the contrary, the place of TARE in BCLC B/C HCCs and, more specifically, in large (>8 cm), multifocal HCCs or in HCCs with portal vein tumor thrombus (PVTT) is highly debated and pathological data in this context are lacking.
At intermediate stage and in the absence of level evidence for overall survival (OS) improvement with TARE [vs. transarterial chemoembolization (TACE)], TACE is still the therapeutic option favored by BCLC and American Association for the Study of Liver Diseases (AASLD) on account of its wider availability (6). Yet, randomized-controlled trials (RCT) (7-9) established a longer time to progression (TTP) and an improved safety and quality of life after TARE vs. TACE.
In case of PVTT, TARE is not recommended because of negative phase III RCTs in BCLC C stage. Several explanations have been put forward to account for such negative results, among which: a poor selection of patients, a learning curve achieved through these trials and the absence of modern dosimetry, in contradiction with basic principles of radiation therapy (10,11). The DOSISPHERE-01 multicenter RCT has recently refocused attention on TARE by demonstrating the superiority of personalized dosimetry over standard dosimetry used in prior negative phase III trials. At interim analysis, the primary endpoint (objective response) was met (71% vs. 36%, P=0.0074) despite the inclusion of poor-prognosis tumors (mean size >10 cm, PVTT in 2/3 cases). Median OS was also more than doubled with personalized dosimetry (26.5 vs. 10.7 months, P=0.0096) and it far exceeded the OS following systemic treatments, albeit recommended. Based on pre-treatment single photon emission computed tomography (SPECT)-CT, personalized dosimetry aimed at delivering high tumor-absorbed dose while keeping >30% hepatic reserve (i.e., untreated liver) for safety purposes.
Surgical treatment of BCLC B or C generally remains controversial (12). Moreover, resection of large, multifocal or PVTT HCC frequently implies a major hepatectomy, thereby raising the additional issue of insufficient future liver remnant (FLR). All this may explain why TARE is mainly considered as a palliative treatment with no hope of cure in these tumors. Consequently, little is known in this poor prognosis population about the pathological response and patient’s outcome after surgery following Yttrium-90 (Y90) downstaging. In DOSISPHERE-01, eleven patients with unresectable HCC (n=8/11 with PVTT) were downstaged by Y90 and eventually underwent surgery (91% R0). The accumulated experience of radiation segmentectomy and lobectomy in early tumors revealed that Y90 allows tumor control, contralateral hypertrophy and an embedded test of time to prevent surgery in patients with aggressive tumor biology (2,13). Taken collectively, these data suggest a potential role of Y90 as a downstaging strategy, even in HCC patients with PVTT, multifocal or large tumors.
The aim of this study was to evaluate safety, radiological and pathological response and outcomes in HCC patients with PVTT, multifocal or large tumors, who underwent surgical treatment after Y90 TARE using personalized dosimetry. We present this article in accordance with the STROBE reporting checklist (available at https://hbsn.amegroups.com/article/view/10.21037/hbsn-22-184/rc)
Methods
Patient selection
Consecutive patients who underwent TARE between December 2015 and October 2021 were included. The study was conducted in accordance with the Declaration of Helsinki (as revised in 2013). This retrospective study was approved by our institutional review board (IRB-UH Montpellier; accreditation number: 198711 and the IRB ID is 202100933) and written informed consent was obtained from all patients. Inclusion criteria were: HCC diagnosed by histopathology or by the imaging criteria of the European Association for the Study of the Liver (EASL); patients treated by TARE using Y90-loaded glass microspheres for a large (i.e., >8 cm) and/or multifocal (all >2 cm to ensure reliable assessment of both imaging response and tumor-absorbed dose) tumor, and/or presenting PVTT; patient who underwent surgical resection or liver transplantation (LT). Exclusion criteria were: patients undergoing additional treatment (either systemic or liver-directed therapy) in the treated zone to capture the pure effects of TARE at pathology; extra-hepatic disease.
In our tertiary center, TARE is indicated in BCLC A patients with non-resectable ≥5 cm HCC, in BCLC B patients or in BCLC C patients with PVTT. In addition, the following criteria had to be fulfilled: preserved liver function (Child-Pugh < B7), unilobar disease with sufficient hepatic reserve (>30%), ECOG 0–1, normal bilirubin level (<2 mg/dL), absence of severe renal impairment [glomerular filtration rate (GFR) <30 mL/min/1.73 m2] and absence of extrahepatic spread. Our policy granted the exception to bilobar disease when it was predominant in one lobe and thermal ablation could efficiently cure the contralateral disease. TARE either served as a bridge/downstaging therapy to surgery or as a palliative treatment in inoperable patients.
TARE was validated by a multidisciplinary tumor board dedicated to liver tumors, including interventional radiologists, hepatologists, oncologists and liver surgeons. Over the study period, our policy was to systematically consider surgery after a test-of-time period ≥3 months, provided enough FLR [standardized FLR (sFLR) ≥40%] and tumor control were achieved. LT was considered only when the tumor was downstaged without any other tumor occurrence for at least 6 months and after external experts appointed by the biomedicine agency in France had given their agreement.
Pretreatment and radioembolization technique
All patients underwent angiography and 99m-Technetium macroaggregated albumin (MAA) SPECT-CT before TARE with Y90 glass microspheres to ensure complete tumor (and PVTT, when present) targeting, while preserving as much nontumoral parenchyma as possible. The lung shunt fraction was determined, extrahepatic MAA distribution was checked and the activity required was calculated using the Medical Internal Radiation Dosimetry (MIRD) method to deliver at least 205 Gy to the tumor and >250–300 Gy if possible, while maintaining <150 Gy to whole liver, in accordance with the personalized dosimetry concept (14). A Y90-positron emission tomography (PET)/CT was systematically performed within 24 hours following TARE.
The pre- and post-treatment absorbed doses were determined from pre-TARE SPECT/CT and post-TARE Y90-PET/CT. In case of tumor multifocality, the absorbed dose delivered to each nodule was estimated for a per-tumor analysis, whereas the lowest tumor-absorbed dose among tumor nodules was recorded for a per-patient analysis.
Baseline imaging and follow-up
Baseline imaging was performed less than one month before TARE and clinical, biological and imaging [CT or magnetic resonance imaging (MRI)] follow-up was conducted every 3 months thereafter. Adverse events related to TARE were classified according to the CTCAE 5.0 grading. Imaging review was carried out by two readers to assess imaging response upon modified response criteria in solid tumors (mRECIST), with discrepancies adjudicated by consensus. As in the LEGACY study (2), we dichotomized localized mRECIST, defined as the tumor response within the treated zone, and mRECIST, which assessed localized tumor response but also distant tumor progression.
PVTT and hepatic vein tumor thrombus (HVT) were classified as described elsewhere (15). Since PVTT/HVT are considered non-measurable upon mRECIST, we considered: (I) complete response (CR) both when tumor response (upon mRECIST) outside PVTT/HVT was complete and when PVTT displayed unenhancement on follow-up images, without any increase in its diameter and without any proximal extension; (II) progressive disease either when progression was noted (upon mRECIST) outside PVTT/HVT, or when increased diameter or extension of a contrast-enhanced PVTT was encountered; (III) partial response (PR) or stable disease (SD), as defined by mRECIST for tumor assessment outside PVTT/HVT, together with the absence of both diameter increase and proximal extension of the PVTT. The same principles were applied to localized mRECIST.
In this study, imaging response on the last follow-up preceding surgery and the best response between TARE and surgery were recorded.
Surgery
sFLR volumetric evaluations were determined using CT/MRI scans, as described elsewhere (16). Surgery was performed by experienced (10 to 20 years) liver surgeons working at our tertiary transplant center. Time from TARE to resection or transplantation, 90-day mortality, morbidity according to Dindo-Clavien classification (17) and post hepatic liver failure (PHLF) according to International Study Group of Liver Surgery (ISGLS) criteria (18) were reported.
Pathology analysis
The explant was evaluated from archived slides by one experienced (>10 years in liver pathology) pathologist for evidence of gross and histological necrosis. After formalin fixation, the resected liver was examined using 4–6 mm slice thickness. For the per-tumor analysis, the percentage of necrosis was reported for each nodule and classified into three groups as previously described (3-5,19): complete pathological necrosis (CPN), extensive (50–99%) and partial (<50%) tumor necrosis. For the per-patient analysis, the poorest pathological response was considered in case of tumor multifocality. The resection margin, the degree of tumor differentiation (poor, moderate or well differentiated) and the presence of microscopic vascular emboli were also evaluated.
Statistical analysis
Continuous variables were described using means ± standard deviation or median and range. Median [and 95% confidence interval (CI)] follow-up was calculated using the reverse Kaplan-Meier method. Progression-free survival (PFS) was defined as the time from TARE to the first recurrence, death or last follow-up. OS was defined as the interval between TARE and death (any cause) or last follow-up. Survival curves were estimated using the Kaplan-Meier method and compared with the log-rank test. A robust variance estimator was used systematically. All the analyses were performed with the Stata software, version 16.1 (Stata corporation, College Station, TX, USA). A P value <0.05 was considered significant.
Results
Population (Figure 1)
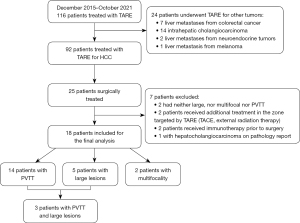
Over the study period, 116 patients underwent TARE with Y90 glass microspheres. Ninety-two had HCC, among whom 25 (27%) were surgically-treated (22 resections and 3 LT). The main reasons for unresectability included progressive disease after TARE, severe portal hypertension precluding resection, severe renal, respiratory or cardiac impairment, advanced age and patient’s refusal. Two surgically-treated patients did not meet the inclusion criteria because they had neither PVTT, nor large or multifocal HCC. Four patients were excluded because they had received additional treatments prior to surgery. One patient was diagnosed with hepatocholangiocarcinoma on the final pathology report and was therefore excluded.
Overall, 18 patients were included in our series. Baseline patient, tumor and treatment characteristics are summarized in Table 1. Fourteen (78%) had PVTT, 2 (11%), multifocal disease and 5 (28%) large lesions. One multifocal patient had 2 tumors (with a sum of diameters of 82 mm) and the other, 3 tumors (with a sum of diameters of 110 mm). Overall, 83% (15/18) of the population had macrovascular invasion (PVTT or HVT). Three patients with PVTT also had large (>8 cm) tumors. No patient with multifocality had PVTT or large lesions. Three patients (17%) had HVT, which was associated with PVTT in 2/3 patients. A total of 22 tumors were available for per-tumor analysis. One patient had bilobar disease but received percutaneous thermal ablation in the contralateral lobe concurrently to TARE.
Table 1
Variables | Values |
---|---|
Age (years) | 68 [49–85] |
Sex | |
Male | 16 [89] |
Female | 2 [11] |
Underlying liver disease | |
HVC/HVB | 4 [22] |
OH | 7 [39] |
NASH | 6 [33] |
No liver disease | 1 [6] |
AFP (ng/mL) | 16 [2–3,071] |
Child-Pugh score | |
A5 | 16 [89] |
A6 | 2 [11] |
MELD score | 7 [6–16] |
ECOG | |
0 | 9 [50] |
1 | 9 [50] |
BCLC stage | |
A | 1 [6] |
B | 3 [17] |
C | 14 [78] |
Treatment before TARE | |
TACE† | 1 [25] |
TA + TACE‡ | 1 [25] |
Systemic treatment | 1 [25] |
Segmentectomy | 1 [25] |
Index tumor size (mm) | 53 [20–150] |
Tumor distribution | |
Unilobar | 17 [94] |
Bilobar | 1 [6] |
PVTT type (15) | |
VP4 | 1 [7] |
VP3 | 5 [36] |
VP2 | 7 [50] |
VP1 | 1 [7] |
HVTT type (15) | |
VV1 | 2 [67] |
VV2 | 1 [33] |
PVTT or HVTT | 15 [83] |
The data are expressed as n [%] or median [range]. †, TACE was performed 4 years before TARE and TARE was performed outside the initial TACE anatomical territory; ‡, TACE was performed 2 years before TARE and TARE was performed outside the initial TACE anatomical territory. TARE, transarterial radioembolization; HVC, hepatitis C; HVB, hepatitis B; OH, alcoholic cirrhosis; NASH, non-alcoholic steatohepatitis; AFP, alpha fetoprotein; MELD, model for end-stage liver disease; ECOG, eastern cooperative oncology group; BCLC, Barcelona clinic liver cancer; TACE, transarterial chemoembolization; TA, thermal ablation; PVTT, portal vein tumoral thrombus; HVTT, hepatic vein tumor thrombus.
TARE and related events
Most patients (13/18) received lobar TARE [right (n=10), left (n=3)], and the remaining patients underwent sectorial and segmental TARE for two and three of them, respectively. Baseline total tumor volume was 82 mL (range, 15–1,769 mL) and baseline perfused-liver volume was 677 mL (range, 265–2,331 mL). A discrepancy between pre-treatment SPECT-CT estimated dosimetry and post-treatment PET-CT results was noticed in two patients: one had a preferential flow in the non-tumor-bearing segment due to vasospasm, which resulted in a poor tumor dose on the post-treatment PET-CT. The other patient had less radiation delivered to the proximal portion of the portal vein thrombosis without any technical explanation. TARE-related adverse events were all mild and transient (Table S1). No radioembolization-induced liver disease (REILD) or pneumonitis was observed. Baseline and pre-operative data on biochemical liver function tests are collected in Table S2.
Tumor response
Localised mRECIST
Seven (39%) patients achieved CR prior to surgery, whereas 10 (56%) achieved PR and 1 (6%) had SD. Response rates were identical when considering the last follow-up preceding surgery and the best response between TARE and surgery. The median time to achieve best response was 3 months (range, 2–11 months).
mRECIST
CR prior to surgery was noted in 7/18 (39%) patients, PR in 8/18 (44%), SD in 1/18 (6%) patients and progressive disease in 2/18 (11%) patients. Progressive disease occurred in the untreated zone and was accessible to a curative treatment. Both patients received intraoperative thermal ablation during surgery. Considering the best response between TARE and surgery, CR was noted in 7/18 (39%) patients, PR in 10/18 (56%) patients and SD in 1/18 (6%) patient.
Median baseline alpha fetoprotein (AFP) was 16.2 ng/mL (range, 2.2–3,071 ng/mL) and AFP 3 months after TARE was 12.9 ng/mL (range, 1.7–85 ng/mL) [P= not significant (NS)]. Data specific to patients with PVTT are summarized in Table S3.
Surgery
Eleven patients underwent major hepatectomy (Brisbane classification), 2 received LT and 5 patients had less extended resections. In patients who underwent major hepatectomy, volumetric evaluations showed a median sFLR of 48% (range, 36–70%) at baseline and 67% (range, 48–107%) the week preceding surgery, which determined a median FLR hypertrophy of 36% (range, 4–81%).
The median time from TARE to surgery was 6.2 months (range, 2–14.6 months). The most common treatment was right hemi-hepatectomy (11/18). The other patients underwent left lobectomy (n=3), segmentectomy (n=1), bi-segmentectomy with additional wedge resection (n=1) and LT (n=2). Parenchymal dissections were performed using CUSA Dissectron® (Integra Life Sciences Corp., Tokyo, Japan) in all cases of resection. Of note, the transplanted patients both presented PVTT at baseline. Median surgery time was 406 minutes (range, 232–672 minutes). Perioperative data are collected in Table 2. Overall, 33% (n=6) ≥ grade III Dindo-Clavien complications were recorded. Two grade B PHLF occurred with full recovery after diuretic therapy. No 90-day mortality was observed.
Table 2
Surgical data | Values |
---|---|
Clavien-Dindo classification | |
3b | |
Hemorrhagic complications requiring reintervention under GA | 1 [6] |
Post-operative wound requiring reintervention under GA | 1 [6] |
Post-operative wound dehiscence | 1 [6] |
Biloma requiring intervention under GA | 2 [12] |
4b (hemorrhagic shock) | 1 [6] |
Blood loss (mL) | 700 [300–2,000] |
90-day mortality | 0 |
PHLF | |
Absent | 14 [78] |
Grade A | 0 |
Grade B (clinical ascites) | 2 [11] |
Grade C | 0 |
Not applicable (liver transplantation) | 2 [11] |
Hospital stays (days) | 12 [7–51] |
ICU stays (days) | 6 [1–25] |
The data are expressed as n [%] or median [range]. GA, general anaesthesia; PHLF, post hepatectomy liver failure; ICU, intensive care unit.
Pathological results
All patients had R0 resection. In per-tumor analysis (n=22), CPN, extensive necrosis and partial necrosis were achieved respectively in 8 (36%), 10 (45%) and 4 (18%) tumors. In per-patient analysis, CPN, extensive necrosis and partial necrosis occurred respectively in 28%, 50% and 22% of patients. Microscopic vascular emboli were reported in 6/18 (33%) patients. Evaluation of cellular differentiation was possible in 12/18 patients (5 had no residual viable tumor cells and one presented extensive necrosis devoid of viable cells for tumor grading). Tumors were well-, moderately-, and poorly-differentiated in 1, 5, and 6, respectively.
Median tumor size was 37 mm (range, 20–93 mm) in tumors showing CPN, 65 mm (range, 34–111 mm) in those with extensive necrosis and 56 mm (range, 29–150 mm) in case of partial necrosis (P=0.24). Baseline AFP levels were highest in patients with CPN with a median of 38 ng/mL (range, 3.5–3,071 ng/mL) (P=0.7). Median AFP levels in patients with extensive and partial necrosis were 15 ng/mL (range, 2.2–1,032 ng/mL) and 16 ng/mL (range, 3.4–16.7 ng/mL) respectively (P=0.7). All patients presenting CPN had PVTT at baseline. In PVTT patients, 36% (5/14) exhibited CPN and none presented microscopic vascular emboli on the explant. Of note, 79% (11/14) exhibited CPN of the PVTT.
Dosimetric considerations
The whole liver-absorbed dose was 99 Gy (range, 30–150 Gy). Post-treatment perfused-liver dose was 202 Gy (range, 123–650 Gy). In per-tumor analysis, the median pre- and post-treatment tumor-absorbed doses were 386 Gy (range, 169–967 Gy) and 331 Gy (range, 31–1,131 Gy) respectively. Tumor-absorbed doses were greater in patients presenting CPN (Table 3). Post-treatment tumor-absorbed dose significantly differed in tumors that exhibited complete (446 Gy), extensive (320 Gy) and partial (187 Gy) pathological necrosis (P=0.045) (Figure 2).
Table 3
Variables | CPN | Extensive | Partial | P value |
---|---|---|---|---|
Tumor absorbed-dose (Y90 PET-CT) | 446 [293–1,131] | 320 [169–678] | 187 [31–340] | 0.045* |
Tumor absorbed-dose (MAA SPECT-CT) | 458 [295–967] | 363 [205–615] | 400 [221–414] | 0.320 |
The data are expressed as median [range]. *, P<0.05. CPN, complete pathological necrosis; Y90, yttrium 90; PET, positron emission tomography; CT, computed tomography; MAA, macro-aggregated albumin; SPECT, single photon emission computed tomography.
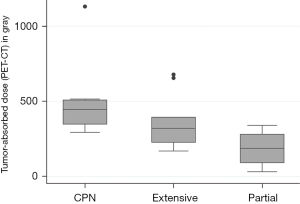
Radiologic-pathologic correlation (Table 4)
Table 4
Variables | Complete response | Partial response | Stable disease |
---|---|---|---|
CPN (n=5) | 5 [100] | 0 | 0 |
Extensive (n=9) | 2 [22] | 7 [78] | 0 |
Partial (n=4) | 0 | 3 [75] | 1 [25] |
The data are expressed as n [%]. mRECIST, modified response evaluation criteria in solid tumors; CPN, complete pathological necrosis.
All patients (5/5) with CPN had CR according to best mRECIST response. Conversely, 71% (5/7) of patients with CR according to best mRECIST response achieved CPN. The remaining patients (2/7) with CR had extensive tumor necrosis at pathology.
Time to surgery and pathological necrosis
Median time from TARE to surgery was 8.3 months (range, 5.8–14.6 months) in patients with CPN, 4.3 months (range, 3.7–13.6 months) in patients with extensive necrosis and 4.9 months (range, 2.1–10.5 months) in patients with partial necrosis. Delay from TARE to surgery was longer in CPN patients (P=0.05).
Patient’s outcome (Figure 3)
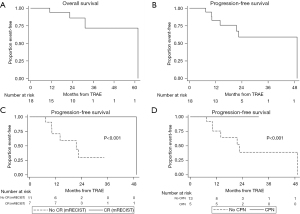
During a median follow-up of 29.6 months (95% CI: 15.4–32.9 months), 4/18 patients died and 7/18 (39%) patients recurred, which determined a median PFS of 49.3 months (95% CI: 14 months–NR) and a median OS of 61.8 months (95% CI: 31.4 months–NR) (Table 5). PFS at 1 and 2 years were 82.6% (95% CI: 55.3–94.1%) and 58.9% (95% CI: 29.3–79.6%), respectively. OS at 1 and 2 years were 93.8% (95% CI: 63.2–99.1%) and 85.9% (95% CI: 54–96.3%), respectively. HCC-related mortality was 1/18. Relapse location and the outcomes of recurring patients are summarized in Table 6. Causes of death in non-relapsing patients were: sudden cardiac arrest (1 patient, 7 months after LT) without any tumor relapse, severe cryptococcal meningitis (1 patient, 7 months after surgery) and septic choc (1 patient, 16.8 months after surgery).
Table 5
Patient | PVTT (15) | HVT (15) | Baseline AFP (ng/mL) | Index tumor size (mm) | Best mRECIST response† | PN | MVE | Post-treatment tumor-absorbed dose (Gy) | TTS (months) | TTR (months) |
---|---|---|---|---|---|---|---|---|---|---|
1 | VP2 | – | 15.6 | 89 | CR | Extensive | 1 | 225 | 13.6 | 49.3 |
2 | VP4 | – | 44.2 | 111 | PR | Extensive | 0 | 169 | 8 | 21.5 |
3 | VP2 | – | 3,071 | 93 | CR | CPN | 0 | 304 | 5.8 | – |
4 | VP3 | – | 1,032 | 50 | PR | Extensive | 1 | 186 | 4.2 | – |
5 | VP2 | – | 3.4 | 32 | SD | Partial | 0 | 31 | 2.1 | – |
6 | VP3 | – | 7.5 | 47 | PR | Extensive | 0 | 322 | 4.1 | 10.3 |
7 | VP2 | – | 38.4 | 41 | CR | CPN | 0 | 394 | 7.8 | – |
8 | VP1 | VV2 | 72.2 | 42 | CR | CPN | 0 | 388 | 8.3 | – |
9 | VP2 | – | 15.4 | 37 | PR | Extensive | 1 | 396 | 3.7 | 14 |
10 | VP2 | VV1 | 2.2 | 77 | PR | Extensive | 1 | 318 | 7.2 | 22.5 |
11 | – | VV1 | 61 | 37 | PR | CPN | 0 | 515 | 6.2 | 7.4 |
29 | – | Partial | – | 148 | – | – | ||||
34 | – | Extensive | – | 382 | – | – | ||||
12 | VP3 | – | 3.5 | 20 | CR | CPN | 0 | 1,131 | 14.6 | – |
13 | – | – | 16.7 | 26 | PR | CPN | 1 | 497 | 10.5 | – |
56 | – | Partial | – | 340 | – | – | ||||
14 | – | – | 12.5 | 150 | PR | Partial | 1 | 225 | 3.5 | 10.1 |
15 | VP3 | – | 13.1 | 26 | CR | CPN | 0 | 293 | 9.7 | – |
37 | – | CPN | – | 506 | – | – | ||||
16 | – | – | 60 | 91 | CR | Extensive | 0 | 311 | 4.3 | – |
17 | VP3 | – | 13.7 | 55 | PR | Extensive | 0 | 678 | 6.2 | – |
18 | VP2 | – | 69.8 | 74 | PR | Extensive | 0 | 655 | 3.9 | – |
†, best mRECIST response = best localized mRECIST response = localized mRECIST response prior to surgery. PVTT, portal vein tumoral thrombosis; HVT, hepatic vein thrombosis; AFP, alpha fetoprotein; mRECIST, modified response evaluation criteria in solid tumors; CR, complete response; PR, partial response; SD, stable disease; PN, pathological necrosis; MVE, microvascular embolism; TTS, time to surgery; TTR, time to recurrence; CPN, complete pathological necrosis; VP1, tumor thrombus distal to the second-order branches of portal vein; VP2, invasion of the second-order branches of portal vein; VP3, thrombus in the first-order branches; VP4, tumor thrombus in main portal trunk or portal branch contralateral to primarily involved lobe (or both); VV1, tumor thrombus in a branch of the hepatic vein; VV2, tumor thrombus in the main trunk of the hepatic veins.
Table 6
Patients | Relapse location | Treatment | Outcome |
---|---|---|---|
1 | Liver followed by pulmonary and lymph nodes metastases | Segmentectomy, systemic treatment†, followed by supportive care | HCC-related mortality due to rapidly progressive disease |
2 | Simultaneous liver and bone metastases | Bevacizumab and atezolizumab; radiation therapy in the localized bone metastasis | Complete radiologic response |
3 | Cardiac metastasis | Systemic treatment‡ | Awaiting treatment evaluation |
4 | Liver | TACI followed by PTA | Awaiting for LT |
5 | Liver | Sorafenib followed by bevacizumab and atezolizumab | Stable disease |
6 | Liver | LT | Mortality related to a sudden cardiac arrest |
7 | Liver | Supportive care | – |
†, Nexavar followed by Lenmiva; ‡, pembrolizumab due to a synchronous lingual tumor. HCC, hepatocellular carcinoma; TACI, transarterial chemo-infusion; PTA, percutaneous thermal ablation; LT, liver transplantation.
Of note, one patient had a nodular implant in the right ventricle, whose metastatic nature was confirmed on biopsy. Interestingly the patient had an initial right HVT. The two other patients with HVT did not develop lung metastasis after a 27- and 28-month follow-up, although one relapsed within the liver. The non-recurring HVT patient also presented PVTT at baseline but achieved CPN.
All 3 patients with incomplete pathological response on the PVTT recurred. Among 7 patients with microscopic vascular emboli, 57% (4/7) recurred.
Among 7 recurring patients, 86% (6/7) remained eligible either to a new curative treatment or to a systemic therapy, whereas one had supportive care due to old age. Two patients underwent additional surgery upon first relapse (LT and segmentectomy, respectively). Three patients received systemic therapy. One patient underwent transarterial chemo-infusion and thermal ablation and is currently awaiting LT after achieving SD.
Patient’s outcome stratified by imaging and pathological response (Figure 4)
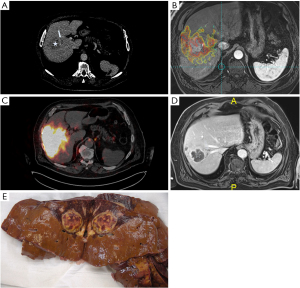
While median PFS was not reached (no recurrence, no death during following) in patients with CPN and in those with CR on imaging, it amounted respectively to 22.5 months (95% CI: 10.1 months–NR) and to 21.5 months (95% CI: 10.1 months–NR) in the others (P<0.001).
Only one patient with CR as best response (1/7; 14%) recurred at 4 years, whereas 55% of patients (6/11) with PR or SD relapsed [median time to recurrence: 1 year (range, 0.6–1.8 years)]. No patients with CPN recurred [median time to recurrence in patients without CPN: 1.2 years (range, 0.6–4.1 years)].
Discussion
The present study shows that successful downstaging of poor-prognosis HCCs can potentially be achieved by Y90 TARE with personalized dosimetry, which yields a high rate of imaging and pathological response. The potential of TARE to successfully bridge/downstage to LT or resection has been widely demonstrated in early HCC, especially through radiation lobectomy (20) and segmentectomy (2). In this context, pathological data have revealed high rates of CPN for small, solitary and treatment-naïve tumors (3-5,19). Our cohort has brought up new data in HCC with PVTT, multifocal disease and large tumors. At baseline, the HCCs in our series were all unresectable and not only outside conventional criteria for LT including the AFP model and Milan criteria, but also outside the extended University of California San Francisco (UCSF) criteria. They were however successfully downstaged using only one single Y90 treatment.
According to AASLD/BCLC (1,21), PVTT patients should undergo systemic therapy, whereas patients with multifocal or large HCCs should be considered for TACE (and optionally for TARE); either for palliative care or as a downstaging strategy to LT. We observed a median OS and PFS of respectively 61.8 and 49.3 months in our cohort, including 78% of patients with PVTT. These survival outcomes far exceed expectations in this poor prognosis population: the results of systemic therapies in this context are considerably worse, as illustrated by the median OS and TTP of respectively 8.1 and 4.1 months after sorafenib (SHARP trial) (22), and by the 14.2-month median OS and the 6.7-month PFS after atezolizumab-bevacizumab (IMBRAVE150 trial) (23). Upfront surgical resection of PVTT HCC usually results in poor outcome (24) and was shown to perform no better than sorafenib (25). Even in expert centers and with progressive refinements in dosimetry, TARE used as a palliative treatment provides a median OS ranging from 16.6 to 32 months in Child-Pugh A patients with branch PVTT (26-29). Therefore, our results suggest an improved outcome of TARE downstaging to surgery over systemic therapy or upfront surgery or TARE alone.
Safety of TARE and surgery
Regarding safety, only mild and transient adverse events were reported in our series after TARE, in keeping with the literature (5,14). As previously reported (20), surgeons could easily differentiate the TARE-treated liver from the untreated liver due to increased inflammation and induration, but they noticed frequent adhesions to adjacent structures which may have complicated surgical resection. Nevertheless, surgery after TARE was safe in our cohort with no 90-day mortality, no grade C PHLF and a 33% rate of ≥ III Dindo-Clavien complications. Perioperative outcomes remained within the range reported for surgical resection without prior TARE (30).
Pathological response
Despite high tumor burden and poor prognosis of our HCC series, CPN occurred after Y90 alone in a significant number of tumors (36%). Of note, all CPN occurred in PVTT HCC, suggesting that PVTT can be completely cured by TARE using personalized dosimetry. Higher rates (47–68%) were reported in pathological series after radiation segmentectomy, but only early HCCs were involved (3-5). Not only did our cohort present frequent PVTT, but the median tumor size was also twice as large (5.3 vs. 2.3–2.6 cm) as that of the previous series. In a series including 5/38 patients with PVTT (19), CPN was reported in 33% of >5 cm tumors, in line with our findings. The larger the tumor, the lower the chance of CPN, due to dose deposition heterogeneity and/or difficulty to reach an efficient dose threshold while keeping safe whole-liver absorbed dose. Not surprisingly, tumors achieving CPN received a significantly greater radiation dose than the others (446 Gy for CPN, 320 Gy and 187 Gy for extensive and partial necrosis respectively, P=0.045) on post-TARE Y90-PET/CT, reflecting the actual dose delivered to the tumor. Similar findings have been reported in T1–T2 solitary (3,5) or T2–T3 (4) HCC.
Achieving CPN after pretransplant locoregional therapies heralds lower recurrence and better survival in LT recipients compared to those exhibiting partial necrosis (31,32). Gabr et al. (20) identified pathological necrosis as a significant prognosticator of recurrence in 31 HCC patients (only one with PVTT) undergoing surgical resection after TARE. In our series of HCC with PVTT, large, or multifocal tumors, no patient with CPN recurred during follow-up, whereas median PFS was 22.5 months in those who did not achieve CPN (P<0.001). Therefore, our findings also support pathological necrosis as a predictor of recurrence in this specific population.
Imaging response
In our series, the best objective response rate (ORR) was obtained at 3 months. While upon localized mRECIST, ORR was high, i.e., 94% (39% CR, 52% PR), it amounted to 83% upon mRECIST (39% CR, 44% PR), thereby confirming the great potential of TARE as a downstaging strategy. A significant correlation between imaging and pathological response has been shown after radiation segmentectomy (22,31,32). Predicting CPN would be highly desirable to avoid unnecessary surgical resection; it is however beyond the scope of our study. Interestingly, all patients presenting CPN after surgery had prior CR on imaging. Conversely, among patients with CR, 71% (5/7) had CPN. Patients with CR on imaging had longer PFS than the others (P<0.001), with only one CR patient recurring at 4 years, whereas 55% of PR/SD patients relapsed at a median time of one year. Taken collectively, these data support referring to surgery the patients exhibiting CR on imaging at 3 months.
Resection or LT after Y90 downstaging
In a very recent study assessing TARE in PVTT patients (n=17) as a downstaging strategy to LT (33), the intention-to-treat analysis showed that only 29% (5/17) actually received LT roughly 2 years after TARE. This long delay is accounted for by the ≥6-month duration of sustained radiological response which is required before patients gain HCC exception points. Among them, 3 had tumor recurrence within 1 year of LT. In this study, a median PFS of 12.1 months after TARE was reported in the whole cohort, 34.6 months in transplanted and 10.3 months in non-transplanted patients. Our results outperform these findings with a 49.1% median PFS and OS rates comparable to those of this study’s transplanted patients, which suggests that resection should be favored whenever possible, keeping LT for non-resectable patients (n=2 in our series) or as salvage therapy, especially in the current context of graft shortage.
Additionally, Serenari et al. (33) raised the ethical issue of the use of deceased donors for HCC patients with macrovascular invasion, given the high recurrence rate [60% in deceased donor liver transplantation (DDLT)] in their cohort. This addresses the important question of appropriate patient selection for surgery. Downstaging/bridging using Y90 TARE basically carries an embedded test of time over several months, particularly useful to select less aggressive tumors (34). In contrast with systemic therapies, the simulation phase based on 99m-Technetium MAA SPECT-CT as part of TARE, provides both an accurate patient selection and a really personalized approach. Interestingly, Y90 also causes contralateral hypertrophy of the untreated FLR during the waiting period, allowing patients to reach safe volumetric thresholds to perform resection (13,35). The median time from radioembolization to surgery was 6.2 months in our cohort, in line with data found in the literature (4,19). The optimal time from Y90 treatment to resection remains debated (35,36), reflecting the complex balance between a higher chance to select patients with limited tumor aggressiveness and higher risks to lose a chance for cure. The existence of a temporal effect between TARE and tumor necrosis has been suggested, a longer time allowing for an optimal cytotoxic effect (4,19). This is also supported by our findings of a longer delay between Y90 and resection in patients presenting CPN vs. the others. Nevertheless, the best tumor response on imaging was noted at 3 months.
Timing for resection after Y90
The nontreated liver volume steadily increased over the 6 months following Y90 TARE, in line with previous reports (13,35). All patients reached safe sFLR volume threshold. Nevertheless, we observed two cases of grade A–B PHLF. This might be explained by discrepancies between liver volume and function, as shown in a recent study on functional changes evaluated on 99m-Tc mebrofenin scintigraphy after Y90 with personalized dosimetry (37). Functional data highlighted a significant decrease of FLR function until 2–3 months after Y90. Such volume/function discrepancy had already been reported in other liver regeneration strategies, such as after portal vein embolization (PVE) (38) or after associating liver partition and portal vein ligation (ALPPS) (39). As after ALPPS, during the first 3 months following Y90, the FLR (i.e., non-treated liver) volume overestimated its function, which could lead to unsafe liver resection caused by insufficient FLR function. Thus, waiting at least 3 months after Y90 would be advisable both to restore baseline whole liver function and to benefit from contralateral functional liver regeneration (37). The optimal test-of-time period should therefore range between 3 to 6 months.
This study is subject to strengths and limitations. The strengths lie first in our reporting on an HCC population with poor prognosis and no hope of cure according to current AASLD and BCLC guidelines. Second, we used pathology results as gold standard by selecting patients treated by Y90 using glass microspheres without concomitant treatment—whether systemic or locoregional—making it possible to capture the pure effects of TARE using personalized dosimetry. Limitations include the retrospective design, the absence of control arm and the small sample size. However, this is the largest study published to date in surgically-treated patients with PVTT, large or multifocal HCC after Y90, with a comprehensive analysis including dosimetric, safety, imaging, pathological and outcome data. Finally, an intention-to-treat analysis was not provided, since only the patients who underwent Y90 TARE and, subsequently, surgery was evaluated.
In conclusion, Y90 TARE using personalized dosimetry can provide high rates of imaging and pathological response in patients with PVTT, large or multifocal HCC. Subsequent surgery is safe and leads to outcomes that far exceed expectations in a population with an otherwise poor prognosis and no chance for cure. This study supports further trials to evaluate the potential benefit in this selected population of Y90 TARE downstaging followed by surgery over currently recommended systemic therapies.
Acknowledgments
The authors thank Sandrine Guinodeau for revising the English.
Funding: None.
Footnote
Reporting Checklist: The authors have completed the STROBE reporting checklist. Available at https://hbsn.amegroups.com/article/view/10.21037/hbsn-22-184/rc
Data Sharing Statement: Available at https://hbsn.amegroups.com/article/view/10.21037/hbsn-22-184/dss
Conflicts of Interest: All authors have completed the ICMJE uniform disclosure form (available at https://hbsn.amegroups.com/article/view/10.21037/hbsn-22-184/coif). FP and BG serve as unpaid editorial board members of Hepatobiliary Surgery and Nutrition. CA and BG report receiving consulting fees from Boston scientific as consultants, and receiving payment from Boston scientific for presentations. The other authors have no conflicts of interest to declare.
Ethical Statement: The authors are accountable for all aspects of the work in ensuring that questions related to the accuracy or integrity of any part of the work are appropriately investigated and resolved. The study was conducted in accordance with the Declaration of Helsinki (as revised in 2013). The study was approved by our institutional review board (IRB - UH Montpellie; accreditation number: 198711 and the IRB ID is 202100933) and written informed consent was obtained from all patients.
Open Access Statement: This is an Open Access article distributed in accordance with the Creative Commons Attribution-NonCommercial-NoDerivs 4.0 International License (CC BY-NC-ND 4.0), which permits the non-commercial replication and distribution of the article with the strict proviso that no changes or edits are made and the original work is properly cited (including links to both the formal publication through the relevant DOI and the license). See: https://creativecommons.org/licenses/by-nc-nd/4.0/.
References
- Reig M, Forner A, Rimola J, et al. BCLC strategy for prognosis prediction and treatment recommendation: The 2022 update. J Hepatol 2022;76:681-93. [Crossref] [PubMed]
- Salem R, Johnson GE, Kim E, et al. Yttrium-90 Radioembolization for the Treatment of Solitary, Unresectable HCC: The LEGACY Study. Hepatology 2021;74:2342-52. [Crossref] [PubMed]
- Gabr A, Riaz A, Johnson GE, et al. Correlation of Y90-absorbed radiation dose to pathological necrosis in hepatocellular carcinoma: confirmatory multicenter analysis in 45 explants. Eur J Nucl Med Mol Imaging 2021;48:580-3. [Crossref] [PubMed]
- Toskich B, Vidal LL, Olson MT, et al. Pathologic Response of Hepatocellular Carcinoma Treated with Yttrium-90 Glass Microsphere Radiation Segmentectomy Prior to Liver Transplantation: A Validation Study. J Vasc Interv Radiol 2021;32:518-26.e1. [Crossref] [PubMed]
- Vouche M, Habib A, Ward TJ, et al. Unresectable solitary hepatocellular carcinoma not amenable to radiofrequency ablation: multicenter radiology-pathology correlation and survival of radiation segmentectomy. Hepatology 2014;60:192-201. [Crossref] [PubMed]
- Marrero JA, Kulik LM, Sirlin CB, et al. Diagnosis, Staging, and Management of Hepatocellular Carcinoma: 2018 Practice Guidance by the American Association for the Study of Liver Diseases. Hepatology 2018;68:723-50. [Crossref] [PubMed]
- Kolligs FT, Bilbao JI, Jakobs T, et al. Pilot randomized trial of selective internal radiation therapy vs. chemoembolization in unresectable hepatocellular carcinoma. Liver Int 2015;35:1715-21. [Crossref] [PubMed]
- Salem R, Gordon AC, Mouli S, et al. Y90 Radioembolization Significantly Prolongs Time to Progression Compared With Chemoembolization in Patients With Hepatocellular Carcinoma. Gastroenterology 2016;151:1155-63.e2. [Crossref] [PubMed]
- Dhondt E, Lambert B, Hermie L, et al. 90Y Radioembolization versus Drug-eluting Bead Chemoembolization for Unresectable Hepatocellular Carcinoma: Results from the TRACE Phase II Randomized Controlled Trial. Radiology 2022;303:699-710. [Crossref] [PubMed]
- Llovet JM, De Baere T, Kulik L, et al. Locoregional therapies in the era of molecular and immune treatments for hepatocellular carcinoma. Nat Rev Gastroenterol Hepatol 2021;18:293-313. [Crossref] [PubMed]
- Salem R, Gabr A, Riaz A, et al. Institutional decision to adopt Y90 as primary treatment for hepatocellular carcinoma informed by a 1,000-patient 15-year experience. Hepatology 2018;68:1429-40. [Crossref] [PubMed]
- Allaire M, Goumard C, Lim C, et al. New frontiers in liver resection for hepatocellular carcinoma. JHEP Rep 2020;2:100134. [Crossref] [PubMed]
- Lewandowski RJ, Donahue L, Chokechanachaisakul A, et al. (90) Y radiation lobectomy: Outcomes following surgical resection in patients with hepatic tumors and small future liver remnant volumes. J Surg Oncol 2016;114:99-105. [Crossref] [PubMed]
- Garin E, Tselikas L, Guiu B, et al. Personalised versus standard dosimetry approach of selective internal radiation therapy in patients with locally advanced hepatocellular carcinoma (DOSISPHERE-01): a randomised, multicentre, open-label phase 2 trial. Lancet Gastroenterol Hepatol 2021;6:17-29. [Crossref] [PubMed]
- Cerrito L, Annicchiarico BE, Iezzi R, et al. Treatment of hepatocellular carcinoma in patients with portal vein tumor thrombosis: Beyond the known frontiers. World J Gastroenterol 2019;25:4360-82. [Crossref] [PubMed]
- Ribero D, Chun YS, Vauthey JN. Standardized liver volumetry for portal vein embolization. Semin Intervent Radiol 2008;25:104-9. [Crossref] [PubMed]
- Dindo D, Demartines N, Clavien PA. Classification of surgical complications: a new proposal with evaluation in a cohort of 6336 patients and results of a survey. Ann Surg 2004;240:205-13. [Crossref] [PubMed]
- Fukushima K, Fukumoto T, Kuramitsu K, et al. Assessment of ISGLS definition of posthepatectomy liver failure and its effect on outcome in patients with hepatocellular carcinoma. J Gastrointest Surg 2014;18:729-36. [Crossref] [PubMed]
- Riaz A, Kulik L, Lewandowski RJ, et al. Radiologic-pathologic correlation of hepatocellular carcinoma treated with internal radiation using yttrium-90 microspheres. Hepatology 2009;49:1185-93. [Crossref] [PubMed]
- Gabr A, Abouchaleh N, Ali R, et al. Outcomes of Surgical Resection after Radioembolization for Hepatocellular Carcinoma. J Vasc Interv Radiol 2018;29:1502-10.e1. [Crossref] [PubMed]
- Heimbach JK, Kulik LM, Finn RS, et al. AASLD guidelines for the treatment of hepatocellular carcinoma. Hepatology 2018;67:358-80. [Crossref] [PubMed]
- Bruix J, Raoul JL, Sherman M, et al. Efficacy and safety of sorafenib in patients with advanced hepatocellular carcinoma: subanalyses of a phase III trial. J Hepatol 2012;57:821-9. [Crossref] [PubMed]
- Cheng AL, Qin S, Ikeda M, et al. Updated efficacy and safety data from IMbrave150: Atezolizumab plus bevacizumab vs. sorafenib for unresectable hepatocellular carcinoma. J Hepatol 2022;76:862-73. [Crossref] [PubMed]
- Torzilli G, Belghiti J, Kokudo N, et al. A snapshot of the effective indications and results of surgery for hepatocellular carcinoma in tertiary referral centers: is it adherent to the EASL/AASLD recommendations?: an observational study of the HCC East-West study group. Ann Surg 2013;257:929-37. [Crossref] [PubMed]
- Costentin CE, Decaens T, Laurent A, et al. Sorafenib vs surgical resection for hepatocellular carcinoma with macrovascular invasion: A propensity score analysis. Liver Int 2017;37:1869-76. [Crossref] [PubMed]
- Garin E, Lenoir L, Edeline J, et al. Boosted selective internal radiation therapy with 90Y-loaded glass microspheres (B-SIRT) for hepatocellular carcinoma patients: a new personalized promising concept. Eur J Nucl Med Mol Imaging 2013;40:1057-68. [Crossref] [PubMed]
- Garin E, Rolland Y, Pracht M, et al. High impact of macroaggregated albumin-based tumour dose on response and overall survival in hepatocellular carcinoma patients treated with 90 Y-loaded glass microsphere radioembolization. Liver Int 2017;37:101-10. [Crossref] [PubMed]
- Spreafico C, Sposito C, Vaiani M, et al. Development of a prognostic score to predict response to Yttrium-90 radioembolization for hepatocellular carcinoma with portal vein invasion. J Hepatol 2018;68:724-32. [Crossref] [PubMed]
- Salem R, Lewandowski RJ, Mulcahy MF, et al. Radioembolization for hepatocellular carcinoma using Yttrium-90 microspheres: a comprehensive report of long-term outcomes. Gastroenterology 2010;138:52-64. [Crossref] [PubMed]
- Gelli M, Sebagh M, Porcher R, et al. Liver Resection for Early Hepatocellular Carcinoma: Preoperative Predictors of Non Transplantable Recurrence and Implications for Treatment Allocation. Ann Surg 2020;272:820-6. [Crossref] [PubMed]
- DiNorcia J, Florman SS, Haydel B, et al. Pathologic Response to Pretransplant Locoregional Therapy is Predictive of Patient Outcome After Liver Transplantation for Hepatocellular Carcinoma: Analysis From the US Multicenter HCC Transplant Consortium. Ann Surg 2020;271:616-24. [Crossref] [PubMed]
- Ravaioli M, Grazi GL, Ercolani G, et al. Partial necrosis on hepatocellular carcinoma nodules facilitates tumor recurrence after liver transplantation. Transplantation 2004;78:1780-6. [Crossref] [PubMed]
- Serenari M, Cappelli A, Cucchetti A, et al. Deceased Donor Liver Transplantation After Radioembolization for Hepatocellular Carcinoma and Portal Vein Tumoral Thrombosis: A Pilot Study. Liver Transpl 2021;27:1758-66. [Crossref] [PubMed]
- Titano J, Noor A, Kim E. Transarterial Chemoembolization and Radioembolization across Barcelona Clinic Liver Cancer Stages. Semin Intervent Radiol 2017;34:109-15. [Crossref] [PubMed]
- Palard X, Edeline J, Rolland Y, et al. Dosimetric parameters predicting contralateral liver hypertrophy after unilobar radioembolization of hepatocellular carcinoma. Eur J Nucl Med Mol Imaging 2018;45:392-401. [Crossref] [PubMed]
- Madoff DC, Odisio BC, Schadde E, et al. Improving the Safety of Major Resection for Hepatobiliary Malignancy: Portal Vein Embolization and Recent Innovations in Liver Regeneration Strategies. Curr Oncol Rep 2020;22:59. [Crossref] [PubMed]
- Allimant C, Deshayes E, Kafrouni M, et al. Hepatobiliary Scintigraphy and Glass 90Y Radioembolization with Personalized Dosimetry: Dynamic Changes in Treated and Nontreated Liver. Diagnostics (Basel) 2021;11:931. [Crossref] [PubMed]
- de Graaf W, van Lienden KP, van den Esschert JW, et al. Increase in future remnant liver function after preoperative portal vein embolization. Br J Surg 2011;98:825-34. [Crossref] [PubMed]
- Olthof PB, Tomassini F, Huespe PE, et al. Hepatobiliary scintigraphy to evaluate liver function in associating liver partition and portal vein ligation for staged hepatectomy: Liver volume overestimates liver function. Surgery 2017;162:775-83. [Crossref] [PubMed]