Influence of immunosuppressive drugs on natural killer cells in therapeutic drug exposure in liver transplantation
Highlight box
Key findings
• Despite an overall comparable immunosuppressive efficiency in terms of prevention of acute rejection, a mammalian target of rapamycin inhibitors (mTORIs)-including regimen might be considered as having less impact on natural killer (NK) cell function.
What is known and what is new?
• We know that mTORI inhibits tumor angiogenesis and cytomegalovirus replication.
• We find that mTORI-including immunosuppression might be considered as having less impact on NK cell function.
What is the implication, and what should change now?
• These findings indicate that the cytolytic activity of NK cells in a mTORI-containing immunosuppression regimen is better preserved than with mycophenolate mofetil and might support the hypothesis of a reduced recurrence rate of hepatocellular carcinoma after liver transplantation.
Introduction
Background
Liver transplantation has become the therapy of choice for various acute and chronic end-stage liver diseases. Since Starzl successfully completed the first human liver transplant in 1963 (1), a total of approximately 500,000 liver transplants have been completed worldwide. After more than half a century of development, the surgical success rate of liver transplantation is also increasing, and the 1-year survival rate after liver transplantation is now approximately 80% (2). The tremendous progress made in the field of liver transplantation has particularly benefited from the emergence of safe and effective immunosuppressive agents. Despite this progress, long-term survival in liver transplant patients is still impaired by interactions between the immune system and the immunosuppressive regimen, such as viral infections or tumor recurrence. Hence, a closer look into the innate immune system and particularly into natural killer (NK) cells under the conditions of the current immunosuppressive therapy is of clinical interest.
Immunosuppression for liver transplantation has seen a steady improvement over the years, leading to widely accepted concepts and standards, including induction therapy and maintenance treatment (3). Major achievements include the characterization of pharmacokinetics and dynamics of calcineurin inhibitors (CNIs), inosine monophosphate dehydrogenase inhibitors, and mTOR inhibitors; minimization concepts of CNIs; and combination concepts of individual drugs to address their individual side effects (3-6).
CNIs are the cornerstone of long-term immunosuppression after liver transplantation (7). The main side effect of CNIs is nephrotoxicity, which can lead to acute kidney injury, and years of cumulative use can lead to chronic renal insufficiency (8,9). Mycophenolic acid inhibits the formation of guanosine monophosphate by blocking inosine monophosphate dehydrogenase (10,11). Used in combination with CNI as a second-line immunosuppressant, it can reduce CNI exposure to prevent nephrotoxicity and can also be used as part of a corticosteroid (CS)-retention regimen (12-14). CS has always been another important part of immunosuppression after liver transplantation, although there is growing evidence that early withdrawal is possible and even beneficial in HCV patients (15). Unfortunately, they have a variety of adverse effects as well, including hyperlipidemia, hypertension, and diabetes (16). Mammalian target of rapamycin inhibitors (mTORIs) are sirolimus (SIR) and everolimus (EVE) (17,18), which are usually delayed by two to four weeks after liver transplantation. Since EVE received approval by the FDA in 2013 (19), its combination with minimized tacrolimus (TAC) exposure is increasingly used. SIR, although not officially approved by the FDA, is widely used in liver transplantation, particularly for patients transplanted for hepatocellular carcinoma (HCC).
Owing to long-term exposure to immunosuppressive drugs, malignant tumors after transplantation, whether de novo or recurrent, are usually more invasive than those in a non-transplant environment. Despite following the Milan or UCSF criteria, the recurrence rate of HCC after liver transplantation is still 8–20% (20,21). Improved immunosuppression management reduces rejection and facilitates long-term graft survival. CNIs such as TAC and CSA are essential for this improvement, but they have several side effects, including an increased cancer risk (22). CNI, which are presently the basis of LT immunosuppressive regimens, have been proven in vitro and in animal models to increase tumor growth and progression through overexpression of transforming growth factor β (23). A higher CNI in the first month after liver transplantation (TAC >10 ng/mL and CSA >300 ng/mL) doubled the chance of HCC recurrence, according to a study based on a cohort of transplanted patients with hepatocellular carcinoma under the Milan criteria (24). Similarly, CS has been found to encourage tumor recurrence. A retrospective analysis showed that CS increased the severity of HCV recurrence by affecting viral load and receptor immune response (25).
mTOR inhibitors have a dual role in providing sufficient immunosuppression to prevent organ rejection and reduce the hazard of de novo malignancy after transplantation. mTORI-mediated tumor inhibition appears to be based on antiangiogenic activity, which is related to impaired vascular endothelial growth factor production and blockade of vascular endothelial cell stimulation induced by vascular endothelial growth factor (26). With abnormal activation of the mTORI pathway in HCC, mTORI can induce vascular remodelling and damage HCC growth (27-30). These findings support the importance of the mTOR pathways in cancer pathogenesis. Hence, in liver transplantation, a combination of CNI with mTOR inhibitors has evolved as an alternative to a combination with mycophenolate mofetil (MMF) for three reasons: (I) reduce the nephrotoxicity of CNI (31-34); (II) reduce the risk of HCC recurrence (35-37); and (III) benefit from its antiviral potency (38).
NK cells are usually considered an integral part of innate immune defense owing to the lack of antigen-specific cell surface receptors (39). NK cells account for 25–50% of the total number of liver lymphocytes (40-42) and are considered to be main regulators in alloimmune reactivity as well as viral and tumor surveillance (43-45). The activation of NK cells depends on the delicate balance between activated receptor-mediated and inhibitory receptor signals. Inhibitory receptors programmed death-1 (PD-1), T-cell immunoreceptor with Ig and ITIM domains (TIGIT), sialic acid-binding immunoglobulin-like lectins (Siglecs), and T-cell immunoglobulin domain and mucin domain-3 (TIM-3) were confirmed to be expressed on NK cells (46). However, the effects of immunosuppressants on NK cells are not clearly understood, and, in particular, the typical clinical exposure regarding combinations and trough levels has not yet been analysed. We present this article in accordance with the TREND reporting checklist (available at https://hbsn.amegroups.com/article/view/10.21037/hbsn-22-438/rc).
Rationale and knowledge gap
The purpose of this study was to evaluate the impact of trough level conditions of immunosuppressive drugs in typical clinically used triple drug combinations and exposure on human NK-cell activity and function.
Objective
Liver transplant recipients
Thirty-five liver transplant recipients were recruited from the First Affiliated Hospital of University of Science and Technology of China, including 27 males and 8 females. All patients received liver transplants from DBD, DCD, or DBCD donors. All procedures were performed according to international standards as described previously by Guo (47) and in accordance with the Declaration of Helsinki (as revised in 2013). Immunosuppression consisted of basiliximab for induction, Tac (C0 3–8 ng/mL), and CSs rapidly tapered to 5 mg qd by day 8 pod. In addition, patients received 1 g of MMF daily or sirolimus (C0 3–8 ng/mL). Patients exposed to SIR had HCC as the primary diagnosis. The details of all patients are provided in Table 1.
Table 1
Medication | TAC + SIR + CS | TAC + MMF + CS |
---|---|---|
Recipient | 13 | 22 |
Gender | Male [13] | Male [14] |
Female [0] | Female [8] | |
Age (years) | 46.8±6.8 | 43.2±10.6 |
Weight (kg) | 74.8±12.0 | 66.5±14.1 |
Time after transplantation (years) | 1.3±1.0 | 1.8±1.2 |
Diagnosis | HCC [7] | Liver cirrhosis [4] |
Liver cirrhosis [6] | Cirrhosis after hepatitis B [15] | |
Acute liver failure [3] | ||
Rejection | No | No |
Survival | Yes | Yes |
Data are presented as mean ± standard deviation or n. TAC, tacrolimus; SIR, sirolimus; CS, corticosteroid; MMF, mycophenolate mofetil; HCC, hepatocellular carcinoma.
Peripheral blood from volunteers with stable liver function for at least 3 months (Table 1) after liver transplantation was collected for the experiment. Owing to restrictions by the Ethical Board, the amount to be collected was limited to 5 mL. Written informed consent was obtained from each patient included in the study, and the protocol of all study cohorts was approved by the Medical Research Ethics Committee of the First Affiliated Hospital of the University of Science and Technology of China (No. 2021 KY212).
Inclusion criteria:
- Patients undergoing allogeneic liver transplantation for various end-stage liver diseases;
- Age: over 18 years old (including 18 years old);
- At least 3 months after liver transplantation, stable liver function was observed.
Healthy volunteers
Thirty healthy volunteers were recruited, and the study was approved by the medical ethics committee of the First Affiliated Hospital of University of Science and Technology of China.
Inclusion criteria:
- Sex: male and female (nonpregnancy, non-lactating);
- Age: over 18 years old (including 18 years old);
- Healthy and do not take any drugs.
Methods
Cell line
Human chronic myelogenous leukemia cells (K562 cells) (catalog number: 1101HUM-PUMC000039) were purchased from the Cell Bank of the Chinese Academy of Science (Shanghai, China). The first human myeloid leukemia artificially cultured cells, erythroleukemia K562 cells, were derived from the lymphoblasts of a 53-year-old female patient with a chronic myeloid leukemia outbreak. The K562 cell line was maintained in RPMI 1640 medium containing 10% fetal bovine serum (FBS).
Human acute promyelocytic leukemia cells (HL60 cells) (catalog number: TCHu 23) were purchased from the Cell Bank of the Chinese Academy of Science (Shanghai, China). These cells were isolated and established by Collins SJ from the peripheral blood of a 36-year-old White woman with acute promyelocytic leukemia. The HL60 cell line was maintained in RPMI 1640 medium containing 10% FBS.
Isolation of peripheral blood mononuclear cells (PBMCs)
Peripheral blood samples from healthy controls were collected from the University of Science and Technology of China (Hefei, China), and PBMCs were isolated by lymphocyte separation fluid (catalogue number: LTS1077-1, TBD, China) density gradient centrifugation according to the manufacturer’s instructions. Human PBMCs were cultured in RPMI 1640 medium with 10% FBS and 100 IU/mL recombinant human IL-2.
Flow cytometry
Cells were phenotypically analysed using the Beckman Flow Cytometer. To exclude dead cells from the analysis, 7-amino-actinomycin-D was added to the cells prior to acquisition. For 9-color analyses on the BACKMAN, the following conjugated mAbs were combined: CD56-Brilliant Violet 605 (catalog number: 362538, BioLegend, China), CD16-Brilliant Violet 510 (catalog number: 302048, BioLegend, China), CD45-APC-Cyanine 7 (catalog number: 368516, BioLegend, China), CD3-Brilliant Violet 785 (catalog number: 344842, BioLegend, China), NKG2A-FITC (catalog number: 130-113-565, BioLegend, Miltenyi, Germany), CD8-PB450 (catalog number: 562428, BD, USA), CD4-APC (catalog number: 5553492, BD, USA), CD33-PE (catalog number: 555450, BD, USA), IFN-γ-FITC (catalog number: 506504, BioLegend, China), CD107a-PE-Cyanine 7 (catalog number: 561348, BD, USA), and Granzyme B-AF647 (catalog number: 560212, BD, USA). Before 9-color analyses were performed, all conjugates were titrated and individually tested for sensitivity, resolution, and compensation of spectral overlap. Isotype controls were used to define marker settings. The combinations were balanced in fluorochrome combinations to avoid antibody interactions and steric hindrance and to detect dimly expressing populations. Then, the cells were analysed using FlowJo software 10.
Isolation of NK cells
NK cells were purified by negative selection using a human NK-Cell Isolation Kit (Catalogue Number: 130-092-657, Miltenyi Biotec, Germany) according to the manufacturer’s instructions. The purity of NK cells was >90%, as determined by flow cytometry. Purified NK cells were cultured in RPMI 1640 medium with 10% FBS and 100 IU/mL recombinant human IL-2.
Cytolytic assay
Cytolytic activity of NK cells to lyse K562 or HL60 cells was detected using the CFSE (Catalogue Number: 565082, BD, USA) staining method. The enriched NK cells from PBMCs of healthy volunteers were exposed to single immunosuppressants (EVE, 5 ng/mL; SIR, 5 ng/mL; TAC, 5 ng/mL; CSA, 125 ng/mL; MMF, 15 µg/mL; CS, 0.5 µg/mL) and combined immunosuppressants (Group 1: TAC, 5 ng/mL + MMF, 15 µg/mL + CS, 0.5 µg/mL; Group 2: TAC, 5 ng/mL + SIR, 5 ng/mL + CS, 0.5 µg/mL; Group 3: TAC, 5 ng/mL + EVE, 5 ng/mL + CS, 0.5 µg/mL) for 3 d; the CTLs did not receive any drugs. NK cells were used as effector cells. K562 cells and HL60 cells grown in logarithmic phase were selected as target cells. The number of target cells was 20,000/well. NK cells and K562 cells or HL60 cells were coincubated for 4.5 h according to the effect target ratios of 2.5:1, 5:1, and 10:1. The ratio of CFSE and DAPI (catalogue number: D1306, Thermo Fisher, USA) double-positive cells was detected by flow cytometry.
RNA extraction
RNA is often isolated and purified from tissues and cells when studying gene expression and regulation. NK cells were isolated from PBMCs of healthy volunteers and exposed to immunosuppressants for 3 d. Then, RNA from NK cells was extracted with TRIzol reagent (catalogue number: 15596018, Ambion, USA) and sequenced.
Bulk RNA sequencing
The research object of transcriptome sequencing (RNA-seq) is the sum of all RNA that can be transcribed by a specific cell in a certain functional state, mainly including mRNA and noncoding RNA. Transcriptome sequencing was based on the Illumina sequencing platform. The technological process of transcriptome sequencing mainly includes sample preparation, library construction, DNA cluster amplification, high-throughput sequencing, and data analysis. RNA-seq was analysed using R 4.1.3 software to focus on gene expression.
The analysis of differential gene expression was carried out using DESeq2. The screening of differentially expressed genes mainly referred to the fold change and q value (Padj, corrected P value). In this study, the significantly differentially expressed genes were screened with | log2 fold change | ≥1 and q<0.05, and the differentially expressed genes were analysed and annotated with Gene Ontology (GO) and Kyoto Encyclopedia of Genes and Genomes (KEGG).
Culture conditions
PBMCs were cultured in the presence of rhIL-2 (100 U/mL) (catalogue number: 200-02, PeproTech, USA) in RPMI 1640 medium (catalogue number: SH30809.01, HyClone, China) supplemented with penicillin (100 U/mL)-streptomycin (100 µg/mL) (catalogue number: SV30010, HyClone, China) and 10% FBS (catalogue number: 10099141, Gibco, Australia) in a 37 ℃, 95% humidity, 5% CO2 incubator. In addition, immunosuppressants were added during the culture for 3 d. Drug type and concentration: single drugs (EVE, 5 ng/mL, Catalogue Number: HY-10218, MCE, USA; SIR, 5 ng/mL, Catalogue Number: HY-10219, MCE, USA; TAC, 5 ng/mL, Catalogue Number: HY-13756, MCE, USA; CSA, 125 ng/mL, Catalogue Number: A600352-0001, BBI, China; MMF, 15 µg/mL, Catalogue Number: HY-B0199, MCE, USA; CS, 0.5 µg/mL, Catalogue Number: A601187-0005, BBI, China) and typical combinations (Group 1: TAC, 5 ng/mL + MMF, 15 µg/mL + CS, 0.5 µg/mL; Group 2: TAC, 5 ng/mL + SIR, 5 ng/mL + CS, 0.5 µg/mL; Group 3: TAC, 5 ng/mL + EVE, 5 ng/mL + CS, 0.5 µg/mL).
Statistical analysis
All experimental data in this study were statistically analysed using GraphPad Prism v8.0.2 software. To compare only the data between the two groups, nonpaired or paired t-test analysis was used. For pairwise comparisons between multiple groups, one-way analysis of variance (ANOVA) was used. All data in this study are expressed as the standard error of the mean (SEM). When P<0.05, the difference was considered to be statistically significant. *P<0.05, **P<0.01, ***P<0.001, ****P<0.0001, NS, not significant.
Results
Low numbers of NK cells in liver transplant recipients compared to normal controls are most likely attributed to the significant proliferation inhibition of combined drug exposure in vitro
We compared the distribution of peripheral blood lymphocytes between liver transplant recipients and healthy controls. The number and proportion of NK cells in the peripheral blood of liver transplant recipients were significantly decreased compared with those of normal controls (Figure 1A). Flow cytometry showed the gating strategy of NK cells (Figure S1A) and the proportion of NK cells to CD45+ lymphocytes in healthy controls and liver transplantation (Figure S1B).
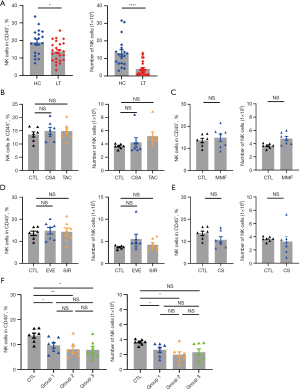
To study the effect of immunosuppressants on PBMCs, we collected PBMCs from healthy people and exposed them to the immunosuppressants commonly used after liver transplantation: CNI (TAC and CSA), MMF, CS, and mTORI (SIR and EVE). The concentration of immunosuppressants in vitro was set according to the clinical effective plasma concentration. Single drug exposure and standard drug combinations were applied. This is the first time the clinical microenvironment of stable liver transplant recipients has been simulated using therapeutic drug plasma concentrations in vitro. There was no significant change in the proportion or number of NK cells and NK cells subgroup (CD56 bright NK cells and CD56 dim NK cells) after exposure to single drugs by flow cytometry (Figure 1B-1E, Figure S2A,S2B). Flow cytometry showed the proportion of NK cells to CD45+ lymphocytes (Figure S2C).
The immunosuppressive effect of the combination regimens was significant for all regimens. The proportion and number of NK cells and NK cells subgroup (CD56 bright NK cells and CD56 dim NK cells) decreased significantly after combination treatment (Figure 1F, Figure S2C-S2E). Under the same conditions, no effect on the number and ratio of CD4 T cells and CD8 T cells was observed, indicating that NK cells react before T cells when the immune system is stimulated (Figure S3A-S3D). Flow cytometry showed the gating strategy of CD4+ T cells and CD8+ T cells (Figure S1A).
CNIs significantly inhibited IFN-γ and CD107a secretion, but no effect on the cytolytic activity of NK cells was observed
Analysis of the activity and function of NK cells after exposure to single immunosuppressants was performed by flow cytometric detection of NKG2D, IFN-γ and CD107a. EVE and CS significantly reduced the expression of the NKG2D receptor in NK cells. Although both EVE and SIR are mTOR inhibitors, EVE was more likely to impair the activity of NK cells (Figure 2A,2B). In addition, CNIs (TAC and CSA), followed by CS, significantly decreased IFN-γ and CD107a, whereas MMF and mTORI did not have a significant effect on NK cells (Figure 2C,2D, Figure S4A).
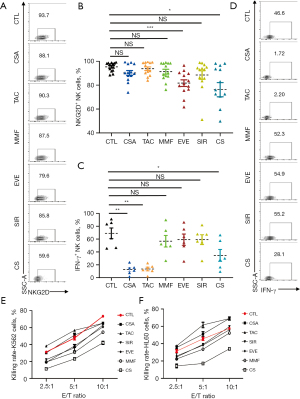
NK cells were sorted from PBMCs, and the yield of NK cells was 91% (Figure S4B). After 3 d of exposure to immunosuppressants, they were incubated with target K562 or HL60 cells, and the cytolytic activity of NK cells was detected by flow cytometry. Flow cytometry showed the gating strategy (Figure S4C). The cytolytic activity increased with the increase in the effective target ratio. When the effect target ratio was 10:1, the cytolytic activity was the highest. CS had the greatest effect on disabling cytolytic activity, followed by MMF and mTORI. Interestingly, CNI (TAC and CSA) had no significant effect on the cytolytic activity of NK cells (Figure 2E,2F), suggesting that IFN-γ and CD107a mediating the cytolytic activity of NK cells on tumor cells does not play a major role. The expression of CD107a on the membrane of CD8+ T cells and NK cells is considered as a marker for activating cytotoxic lymphocytes, indicating that CD107a does not directly affect the cytolytic capacity of NK cells. NK cells act as the early source of IFN-γ and activate T cells, thus triggering a rejection response, while CNIs significantly inhibit IFN-γ secretion by NK cells, which in turn reduces the activation of T cells. This further explains why CNIs have always been the cornerstone of immunosuppression after liver transplantation.
NK-cell function is downregulated by standard immunosuppressive regimens
To better understand the clinical conditions, the immunosuppressive microenvironment was simulated using three standard immunosuppressive regimens (Group 1: TAC + MMF + CS, Group 2: TAC + SIR + CS, Group 3: TAC + EVE + CS). The expression of NKG2D (Figure 3A,3B), IFN-γ and CD107a secretion (Figure 3C,3D, Figure S4D), and tumor cytolytic activity of NK cells (Figure 3E,3F) were significantly downregulated. In addition, the expression of NKG2D decreased after mTOR inhibitor treatment compared with that of NK cells in Group 1 containing MMF (Figure 3B). The expression of NKG2D may be more sensitive to the inhibition of the mTOR signaling pathway.
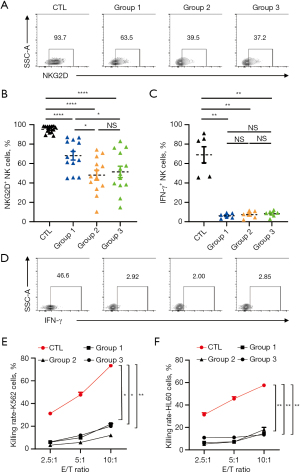
At the gene level, the triple drug regimens demonstrated pronounced inhibition of NK-cell function, lower NK-cell activation and function, and stronger apoptosis and inhibition
To study the effect of immunosuppressants on NK cells at the gene level, we extracted and sequenced the RNA of NK cells after exposure to immunosuppressants. Compared with the expression in the healthy control, all differential genes were taken and collected as the differential gene set. We found that the expression under CS treatment was similar to that of the combined immunosuppressant treatment group, EVE and SIR were mTORI, their expression patterns were similar, and the differential gene expression of CNI, CSA, and TAC were similar (Figure 4A).
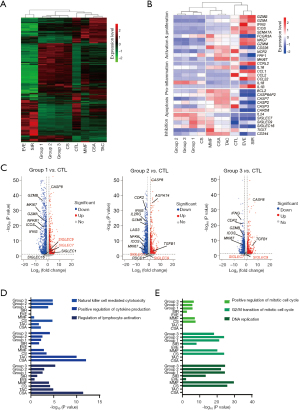
The genes were classified according to five functions: activation, proliferation, proinflammatory, apoptosis, and inhibition (Figure 4B). CS and triple drug combinations significantly reduced the activation and proinflammatory gene set of NK cells, while mTOR inhibition had no significant effect; MMF, mTORI, CS, and combined immunosuppressants downregulated the proliferation gene set of NK cells, while CNI had no significant effect on the proliferation gene set of NK cells; EVE and SIR had no significant effect on NK-cell apoptosis and inhibition. NK-cell apoptosis and inhibition gene expression in the other treatment groups increased to varying degrees.
The combined immunosuppressive therapy groups reflected the real medication status of liver transplant patients. We analysed the differentially expressed genes in the combined immunosuppressive therapy group by volcano map (Figure 4C). As shown in Figure 4C, compared with that in CTL, the expression of activated genes (ICOS, GZMB, GZMA, IFNG), proliferation gene (MKI67), and apoptosis gene (CASP8) in the combination group decreased, which is consistent with the conclusion in Figure 4B. At the same time, we found that the expression of the Siglec family was significantly higher in Group 1 (TAC + CS + MMF) but not in Group 2 (TAC + CS + SIR) or Group 3 (TAC + CS + EVE). Figure 4B also shows that MMF had a stronger inhibitory effect on NK cells than EVE and SIR after single drug action.
GO enrichment analysis of the downregulated differential genes showed that CNIs significantly downregulated NK-cell activation genes, followed by CS and MMF, which was consistent with the previous flow cytometry conclusion (Figure 4D,4E). MMF and CS significantly decreased DNA replication and the cell cycle. SIR had a stronger effect than EVE, whereas TAC and CSA had no effect. According to the results of flow cytometry and sequencing, the triple drug treatment group had the strongest inhibitory effect on NK cells.
NK-cell activity and function were low in liver transplantation patients
Next, we assessed the function of NK cells in vivo. The secretion of the functional molecules IFN-γ, CD107a, and granzyme B decreased, and the expression of the inhibitory molecules NKG2A and CD33 increased (Figure 5A-5E, flow chart on the left and statistical chart on the right), indicating that NK cells in liver transplant recipients were in an inhibitory state.
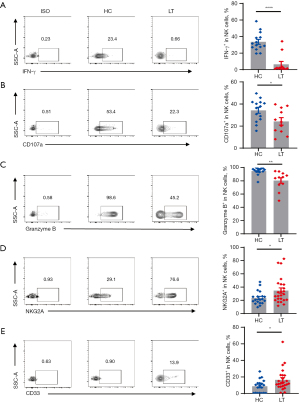
The SIR group had stronger NK-cell activity than the MMF group
As referenced in the methods section, patients with HCC or renal impairment are exposed to SIR, while all other patients receive MMF in combination with TAC and CS. The proportion and number of CD56bright NK cells in liver transplantation increased, while the number of CD56dim NK cells decreased. Because 90% of peripheral blood was in the CD56dim NK-cell subgroup, the number of whole NK cells in liver transplantation was reduced (Figure 6A). The expression of the NK-cell inhibitory receptors NKG2A and Siglec7 increased in liver transplant volunteers taking drugs containing MMF, indicating that MMF can inhibit the activity of the CD56bright subgroup. The expression of the inhibitory receptor of CD56dim in liver transplantation volunteers taking drugs containing SIRs decreased (Figure 6B,6C). In the SIR group, the expression of the inhibitory receptors NKG2A and Siglec7 was lower, and the expression of CD107a was higher (Figure 6D). For the sorted NK cells exposed to MMF and Sir alone, RNA sequencing found that compared with that of MMF, the expression level of activated genes (IL1R2, IL2RA, IL7R, IL17RC, IL17RE, IL23R, CRTAM, GZMA, GZMB, GZMK, and KLRB1) of SIR was higher, and the expression level of inhibitory genes (SIGLEC7, SIGLEC9, SLAMF7, and TIGIT) was lower (Figure 6E). Hence, NK-cell activity and function of the SIR group were stronger and less inhibited, respectively.
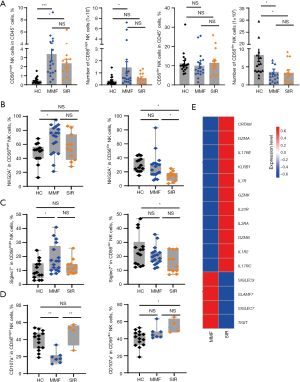
Discussion
Since T cells have long been considered to be the main influencing factor of graft rejection (47,48), the development of immunosuppressive strategies has focused on blocking the activation and proliferation of these cells. Immunosuppressive regimens containing CNIs effectively target recipient T cells, preventing acute rejection, and are the main immunosuppressive agents for liver transplantation (49). At the same time, these regimens are associated with adverse events, such as renal dysfunction, neurotoxicity, and diabetes (50).
Current evidence suggests that NK cells play a critical role in the immune surveillance with regard to viral infections or recognition of tumor cells (i.e., recurrence of HCC). In the particular setting of liver transplant patients, the general function of NK cells under conditions of standard immunosuppression exposure is of relevance to be understood. Of note is to understand not only the individual effect of an immunosuppressive agent on NK cells but in particular the combined effect of the immunosuppressive drugs in long-term stable exposure.
NK cells are the main regulators in liver transplantation regarding rejection, viral infection, or tumor recurrence. In vitro studies have found that CNIs significantly inhibit the secretion of IFN-γ and CD107a by NK cells, thereby reducing T-cell-mediated rejection. In Meehan’s study, CSA showed CD107a expression and IFN-γ production reduction (51). Interestingly, we demonstrated that TAC and CSA had little effect on NK cells’ ability to kill K562 or HL60 tumor cells, implying that CNI had no significant effect on the antitumor effect of NK cells. The downregulated genes of NK cells treated with CNI were considerably enriched in cell activation pathways but not in cell cycle or DNA replication pathways, according to transcriptome sequencing results of NK cells. Although other studies have demonstrated that TAC has a larger inhibitory impact on T-cell activation and is superior to CSA in acute rejection, our findings reveal that the effects of CSA and TAC on NK cells are not significantly different. Based on the above studies, CNIs have the advantages of decreasing T-cell activation caused by NK cells, lowering the risk of graft-versus-host disease, preserving the antitumor ability of NK cells, and not interfering with the NK-cell cycle.
Our data imply that the effect of CS on NK cells is similar to that of a combined immunosuppressive regimen. Its inhibitory effect on NK cells is the most potent among the six immunosuppressive agents studied. CS severely impaired cytolytic and NK-cell activities, inhibited the ability of NK cells to secrete interferon-γ, downregulated the NK-cell cycle, and led to a decrease in the number of NK cells. CS has specific and nonspecific immunosuppressive effects and severely impairs NK cells in their surveillance function regarding viral infections or tumor recurrence. These findings stress why steroid withdrawal is already successfully performed in HCV liver transplant recipients (16,52).
MMF mainly blocks the proliferation of T and B cells (53). Although some studies have shown that the higher the concentration of MMF, the more obvious the damage and inhibition of NK cells (54), but the impact of NK cells exposed to the effective plasma concentration of MMF is unknown. In clinical practice, high dose exposure will be accompanied by side effects, such as gastrointestinal symptoms and dose related bone marrow suppression. Our in vitro experiments have shown that MMF has no effect on the secretion of interferon-γ by NK cells or the number of NK cells but impairs the cytolytic activity of NK cells within a certain range. SIR and EVE are widely used in liver transplantation, although only EVE has FDA and EMA approval. mTORI is preferably used in combination with CNI to improve renal dysfunction and to reduce the risk of tumor recurrence after liver transplantation (3-5). At present, the common clinical protocol after liver transplantation usually adopts the triple immunosuppressive protocol (3): TAC + MMF + CS. For patients with liver cancer and liver transplantation, the clinical protocol is usually converted to TAC + CS + SIR within one month of postoperative time. Our research also confirmed that NK cells treated with SIR or EVE still have a higher cytolytic activity than NK cells treated with MMF. Transcriptome sequencing results showed that after 3 d of exposure to mTORI, NK-cell activation and proinflammatory gene expression did not decrease. Interestingly, as mentioned earlier, the number of NK cells did not decrease, but the decreased expression of the proliferation gene MKI67 indicates that the proliferation of NK cells was affected. We assume that because the expression of NK-cell apoptosis genes was decreased, the total number of NK cells did not change significantly.
To better simulate the actual clinical immunosuppressive microenvironment, we set up three groups of triple immunosuppressive regimens: TAC + MMF + CS, TAC + SIR + CS, and TAC + EVE + CS. The experimental results show that the combination of drugs as expected had the strongest immunosuppressive effect. The number, proportion, activation, cytolytic activity, and proliferation of NK cells were inhibited, and the expression of apoptosis and suppressor genes increased. Compared with the other two groups, the combination containing MMF had a higher degree of suppressive gene expression, especially for the inhibitory receptors Siglec7 and Siglec9 (55-57). Siglec-7 and Siglec-9 ligands are widely expressed on tumor cells of different histological origins and protect tumor cells from NK-cell responses (30). Recently, these immune checkpoints have been shown to participate in the immune escape of various cancers and are therefore becoming an important treatment mode for cancer patients. At the same time, we demonstrated that the expression of NK inhibitory genes after single MMF exposure was higher than that of EVE and SIR. These data suggest that mTORI has a more favorable effect on NK-cell integrity and function under in vivo conditions following liver transplantation. The limitation of this study is still at the gene level, and we still need to carry out functional verification in future research.
In vivo experiments by flow cytometry showed that degranulation (CD107A) and the release of IFN-γ and granzyme B decreased and the expression of inhibitory receptors increased in the peripheral blood of liver transplant recipients. Here, we had two patient groups being treated with MMF or SIR as part of their standard immunosuppression. The expression of the NK-cell suppressor receptor NKG2A was decreased, and the expression of granzyme B was increased under a treatment regimen with SIR, indicating that NK-cell activity and function were better preserved in the SIR group. Our findings are in line with those of Pradier et al. (58), showing that methylprednisolone and EVE as well as SIR induce minor changes in the expression of NKG2D.
The adult human liver is enriched with NK cells, accounting for 30–50% of hepatic lymphocytes, which include tissue-resident hepatic NK-cell subpopulations, distinct from peripheral blood NK cells. Hepatic CD56bright NK cells were Eomeshi T-betlo, a phenotype virtually absent from peripheral blood. These NK cells express the chemokine receptor CXCR6, a marker of tissue residency, which is absent from hepatic CD56dim and blood NK cells. Compared to blood populations, these hepatic CD56bright NK cells have an increased expression of activating receptors (NKp44, NKp46, and NKG2D). They show reduced ability to produce IFN-γ but enhanced degranulation in response to challenge with target cells (59). Hepatic NK cells are of importance in the context of tissue homeostasis, liver infection, malignancy, and transplantation. Due to ethical restrictions, we were unable to obtain liver NK cells from liver transplant recipients, and we could only study the effects of immunosuppressants on peripheral blood NK cells, which is the limitation of this project.
Conclusions
We find that the number and function of peripheral blood NK cells decreased in liver transplant patients. In vitro studies demonstrated that CS seriously impaired the cytolytic activity of NK cells, followed by MMF and mTORI (EVE and SIR). At the same time, CS and CNI seriously impaired the secretion of IFN-γ in NK cells. CS alone had the strongest inhibitory effect on NK cells. As expected, the triple drug regimens demonstrated the most potent immunosuppressive effect on NK cells. Compared with those in the other two groups, the expression of inhibitory genes in the group containing MMF was higher, especially the inhibitory receptor Siglec family. These findings indicate that the cytolytic activity of NK cells in a mTORI-containing regimen is better preserved than in an MMF-containing regimen and might support one potential explanation for the reduced recurrence rate of HCC and the widely observed lower rates of de novo malignancies in patients after transplantation under long-term mTORI exposure.
Acknowledgments
This study was initiated by Professor Björn Nashan with the help of all members of the Organ Transplant Unit and Organ Transplantation & Immunology Laboratory.
Funding: The project was funded by
Footnote
Reporting Checklist: The authors have completed the TREND reporting checklist. Available at https://hbsn.amegroups.com/article/view/10.21037/hbsn-22-438/rc
Data Sharing Statement: Available at https://hbsn.amegroups.com/article/view/10.21037/hbsn-22-438/dss
Conflicts of Interest: All authors have completed the ICMJE uniform disclosure form (available at https://hbsn.amegroups.com/article/view/10.21037/hbsn-22-438/coif). All authors reported that the project was funded by B.N. research start-up fund (2018KYQ003RC2017001), National Natural Science Foundation of China (#8202290021, #92169118, #91942310), National Key Research and Development Program of China (#2021YFC2300600), Anhui Provincial Natural Science Foundation (#2008085J35), and the Fundamental Research Funds for the Central Universities (WK9110000055). B.N. serves as an unpaid editorial board member of Hepatobiliary Surgery and Nutrition. The authors have no other conflicts of interest to declare.
Ethical Statement: The authors are accountable for all aspects of the work in ensuring that questions related to the accuracy or integrity of any part of the work are appropriately investigated and resolved. This study was carried out in accordance with the Declaration of Helsinki (as revised in 2013) and was approved by the Medical Research Ethics Committee of the First Affiliated Hospital of the University of Science and Technology of China (No. 2021 KY212). Informed consent was obtained from all patients included in the study.
Open Access Statement: This is an Open Access article distributed in accordance with the Creative Commons Attribution-NonCommercial-NoDerivs 4.0 International License (CC BY-NC-ND 4.0), which permits the non-commercial replication and distribution of the article with the strict proviso that no changes or edits are made and the original work is properly cited (including links to both the formal publication through the relevant DOI and the license). See: https://creativecommons.org/licenses/by-nc-nd/4.0/.
References
- Starzl TE, Marchioro TL, Vonkaulla KN, et al. Homotransplantation of the liver in humans. Surg Gynecol Obstet 1963;117:659-76.
- Jochmans I, van Rosmalen M, Pirenne J, et al. Adult Liver Allocation in Eurotransplant. Transplantation 2017;101:1542-50. [Crossref] [PubMed]
- Dhanasekaran R. Management of Immunosuppression in Liver Transplantation. Clin Liver Dis 2017;21:337-53. [Crossref] [PubMed]
- Nashan B, Schemmer P, Braun F, et al. Early Everolimus-Facilitated Reduced Tacrolimus in Liver Transplantation: Results From the Randomized HEPHAISTOS Trial. Liver Transpl 2022;28:998-1010. [Crossref] [PubMed]
- Nashan B, Saliba F, Durand F, et al. Pharmacokinetics, efficacy, and safety of mycophenolate mofetil in combination with standard-dose or reduced-dose tacrolimus in liver transplant recipients. Liver Transpl 2009;15:136-47. [Crossref] [PubMed]
- Martinez-Cibrian N, Zeiser R, Perez-Simon JA. Graft-versus-host disease prophylaxis: Pathophysiology-based review on current approaches and future directions. Blood Rev 2021;48:100792. [Crossref] [PubMed]
- Barbier L, Garcia S, Cros J, et al. Assessment of chronic rejection in liver graft recipients receiving immunosuppression with low-dose calcineurin inhibitors. J Hepatol 2013;59:1223-30. [Crossref] [PubMed]
- Jacquet A, Francois H, Frangie C, et al. Prevention of calcineurin inhibitor nephrotoxicity in renal transplantation. Transpl Immunol 2008;20:29-31. [Crossref] [PubMed]
- Issa N, Kukla A, Ibrahim HN. The over-exaggerated chronic nephrotoxicity of calcineurin inhibitors. Arab J Urol 2012;10:169-74. [Crossref] [PubMed]
- Giannakopoulos G, Verbaan H, Friis-Liby IL, et al. Mycophenolate mofetil treatment in patients with autoimmune hepatitis failing standard therapy with prednisolone and azathioprine. Dig Liver Dis 2019;51:253-7. [Crossref] [PubMed]
- Kaltenborn A, Schrem H. Mycophenolate mofetil in liver transplantation: a review. Ann Transplant 2013;18:685-96. [Crossref] [PubMed]
- Lerut J, Bonaccorsi-Riani E, Finet P, et al. Minimization of steroids in liver transplantation. Transpl Int 2009;22:2-19. [Crossref] [PubMed]
- Wang P, Que W, Li H, et al. Efficacy and safety of a reduced calcineurin inhibitor dose combined with mycophenolate mofetil in liver transplant patients with chronic renal dysfunction. Oncotarget 2017;8:57505-15. [Crossref] [PubMed]
- Grinyó JM, Cruzado JM. Mycophenolate mofetil and calcineurin-inhibitor reduction: recent progress. Am J Transplant 2009;9:2447-52. [Crossref] [PubMed]
- Klintmalm GB, Nashan B. The Role of mTOR Inhibitors in Liver Transplantation: Reviewing the Evidence. J Transplant 2014;2014:845438. [Crossref] [PubMed]
- Ramirez CB, Doria C, Frank AM, et al. Completely steroid-free immunosuppression in liver transplantation: a randomized study. Clin Transplant 2013;27:463-71. [Crossref] [PubMed]
- Nashan B. mTOR inhibition and clinical transplantation. Liver Transpl 2018;102:S19-26. [Crossref] [PubMed]
- Cornu M, Albert V, Hall MN. mTOR in aging, metabolism, and cancer. Curr Opin Genet Dev 2013;23:53-62. [Crossref] [PubMed]
- Available online: https://www.drugtopics.com/view/fda-approves-first-drug-over-decade-prevention-organ-rejection
- Ma MKM, Yung S, Chan TM. mTOR Inhibition and Kidney Diseases. Transplantation 2018;102:S32-40. [Crossref] [PubMed]
- Sapisochin G, Bruix J. Liver transplantation for hepatocellular carcinoma: outcomes and novel surgical approaches. Nat Rev Gastroenterol Hepatol 2017;14:203-17. [Crossref] [PubMed]
- Chagas AL, Felga GEG, Diniz MA, et al. Hepatocellular carcinoma recurrence after liver transplantation in a Brazilian multicenter study: clinical profile and prognostic factors of survival. Eur J Gastroenterol Hepatol 2019;31:1148-56. [Crossref] [PubMed]
- Zhu H, Sun Q, Tan C, et al. Tacrolimus promotes hepatocellular carcinoma and enhances CXCR4/SDF-1α expression in vivo. Mol Med Rep 2014;10:585-92. [Crossref] [PubMed]
- Maluccio M, Sharma V, Lagman M, et al. Tacrolimus enhances transforming growth factor-beta1 expression and promotes tumor progression. Transplantation 2003;76:597-602. [Crossref] [PubMed]
- Rodríguez-Perálvarez M, Tsochatzis E, Naveas MC, et al. Reduced exposure to calcineurin inhibitors early after liver transplantation prevents recurrence of hepatocellular carcinoma. J Hepatol 2013;59:1193-9. [Crossref] [PubMed]
- Brillanti S, Vivarelli M, De Ruvo N, et al. Slowly tapering off steroids protects the graft against hepatitis C recurrence after liver transplantation. Liver Transpl 2002;8:884-8. [Crossref] [PubMed]
- Klintmalm GB, Saab S, Hong JC, et al. The role of mammalian target of rapamycin inhibitors in the management of post-transplant malignancy. Clin Transplant 2014;28:635-48. [Crossref] [PubMed]
- Ozturk N, Ozturk D, Pala-Kara Z, et al. The immune system as a chronotoxicity target of the anticancer mTOR inhibitor everolimus. Chronobiol Int 2018;35:705-18. [Crossref] [PubMed]
- Yan X, Huang S, Yang Y, et al. Sirolimus or Everolimus Improves Survival After Liver Transplantation for Hepatocellular Carcinoma: A Systematic Review and Meta-Analysis. Liver Transpl 2022;28:1063-77. [Crossref] [PubMed]
- Grigg SE, Sarri GL, Gow PJ, et al. Systematic review with meta-analysis: sirolimus- or everolimus-based immunosuppression following liver transplantation for hepatocellular carcinoma. Aliment Pharmacol Ther 2019;49:1260-73. [Crossref] [PubMed]
- Saber-Moghaddam N, Nomani H, Sahebkar A, et al. The change of immunosuppressive regimen from calcineurin inhibitors to mammalian target of rapamycin (mTOR) inhibitors and its effect on malignancy following heart transplantation. Int Immunopharmacol 2019;69:150-8. [Crossref] [PubMed]
- Murakami N, Riella LV, Funakoshi T. Risk of metabolic complications in kidney transplantation after conversion to mTOR inhibitor: a systematic review and meta-analysis. Am J Transplant 2014;14:2317-27. [Crossref] [PubMed]
- Shihab F, Christians U, Smith L, et al. Focus on mTOR inhibitors and tacrolimus in renal transplantation: pharmacokinetics, exposure-response relationships, and clinical outcomes. Transpl Immunol 2014;31:22-32. [Crossref] [PubMed]
- Kornberg A, Küpper B, Thrum K, et al. Sustained renal response to mycophenolate mofetil and CNI taper promotes survival in liver transplant patients with CNI-related renal dysfunction. Dig Dis Sci 2011;56:244-51. [Crossref] [PubMed]
- Ferrín G, Guerrero M, Amado V, et al. Activation of mTOR Signaling Pathway in Hepatocellular Carcinoma. Int J Mol Sci 2020;21:1266. [Crossref] [PubMed]
- Duvoux C, Toso C. mTOR inhibitor therapy: Does it prevent HCC recurrence after liver transplantation? Transplant Rev (Orlando) 2015;29:168-74. [Crossref] [PubMed]
- Guerrero M, Ferrín G, Rodríguez-Perálvarez M, et al. mTOR Expression in Liver Transplant Candidates with Hepatocellular Carcinoma: Impact on Histological Features and Tumour Recurrence. Int J Mol Sci 2019;20:336. [Crossref] [PubMed]
- Brennan DC, Aguado JM, Potena L, et al. Effect of maintenance immunosuppressive drugs on virus pathobiology: evidence and potential mechanisms. Rev Med Virol 2013;23:97-125. [Crossref] [PubMed]
- Vivier E, Raulet DH, Moretta A, et al. Innate or adaptive immunity? The example of natural killer cells. Science 2011;331:44-9. [Crossref] [PubMed]
- Robinson MW, Harmon C, O'Farrelly C. Liver immunology and its role in inflammation and homeostasis. Cell Mol Immunol 2016;13:267-76. [Crossref] [PubMed]
- Kalathil SG, Thanavala Y. Natural Killer Cells and T Cells in Hepatocellular Carcinoma and Viral Hepatitis: Current Status and Perspectives for Future Immunotherapeutic Approaches. Cells 2021;10:1332. [Crossref] [PubMed]
- Sun C, Sun HY, Xiao WH, et al. Natural killer cell dysfunction in hepatocellular carcinoma and NK cell-based immunotherapy. Acta Pharmacol Sin 2015;36:1191-9. [Crossref] [PubMed]
- Harmon C, Sanchez-Fueyo A, O'Farrelly C, et al. Natural Killer Cells and Liver Transplantation: Orchestrators of Rejection or Tolerance? Am J Transplant 2016;16:751-7. [Crossref] [PubMed]
- Li L, Wozniak LJ, Rodder S, et al. A common peripheral blood gene set for diagnosis of operational tolerance in pediatric and adult liver transplantation. Am J Transplant 2012;12:1218-28. [Crossref] [PubMed]
- Yuan X, Rasul F, Nashan B, et al. Innate lymphoid cells and cancer: Role in tumor progression and inhibition. Eur J Immunol 2021;51:2188-205. [Crossref] [PubMed]
- Khan M, Arooj S, Wang H NK. Cell-Based Immune Checkpoint Inhibition. Front Immunol 2020;11:167. [Crossref] [PubMed]
- Guo Y. The "Chinese Mode" of organ donation and transplantation: moving towards the center stage of the world. Hepatobiliary Surg Nutr 2018;7:61-2. Erratum in: Hepatobiliary Surg Nutr 2019;8:87. [Crossref] [PubMed]
- Ingulli E. Mechanism of cellular rejection in transplantation. Pediatr Nephrol 2010;25:61-74. [Crossref] [PubMed]
- Ronca V, Wootton G, Milani C, et al. The Immunological Basis of Liver Allograft Rejection. Front Immunol 2020;11:2155. [Crossref] [PubMed]
- Azzi JR, Sayegh MH, Mallat SG. Calcineurin inhibitors: 40 years later, can't live without ... J Immunol 2013;191:5785-91. [Crossref] [PubMed]
- Meehan AC, Mifsud NA, Nguyen TH, et al. Impact of commonly used transplant immunosuppressive drugs on human NK cell function is dependent upon stimulation condition. PLoS One 2013;8:e60144. [Crossref] [PubMed]
- Ohira M, Nishida S, Tryphonopoulos P, et al. Impact of Steroids on Natural Killer Cells Against Cytotoxicity and Hepatitis C Virus Replication. Transplant Proc 2017;49:1160-4. [Crossref] [PubMed]
- Allison AC, Eugui EM. Mycophenolate mofetil and its mechanisms of action. Immunopharmacology 2000;47:85-118. [Crossref] [PubMed]
- Eissens DN, Van Der Meer A, Van Cranenbroek B, et al. Rapamycin and MPA, but not CsA, impair human NK cell cytotoxicity due to differential effects on NK cell phenotype. Am J Transplant 2010;10:1981-90. [Crossref] [PubMed]
- Zheng Y, Ma X, Su D, et al. The Roles of Siglec7 and Siglec9 on Natural Killer Cells in Virus Infection and Tumour Progression. J Immunol Res 2020;2020:6243819. [Crossref] [PubMed]
- Ibarlucea-Benitez I, Weitzenfeld P, Smith P, et al. Siglecs-7/9 function as inhibitory immune checkpoints in vivo and can be targeted to enhance therapeutic antitumor immunity. Proc Natl Acad Sci U S A 2021;118:e2107424118. [Crossref] [PubMed]
- Tao L, Wang S, Yang L, et al. Reduced Siglec-7 expression on NK cells predicts NK cell dysfunction in primary hepatocellular carcinoma. Clin Exp Immunol 2020;201:161-70. [Crossref] [PubMed]
- Pradier A, Papaserafeim M, Li N, et al. Small-Molecule Immunosuppressive Drugs and Therapeutic Immunoglobulins Differentially Inhibit NK Cell Effector Functions in vitro. Front Immunol 2019;10:556. [Crossref] [PubMed]
- Harmon C, Robinson MW, Fahey R, et al. Tissue-resident Eomes(hi) T-bet(lo) CD56(bright) NK cells with reduced proinflammatory potential are enriched in the adult human liver. Eur J Immunol 2016;46:2111-20. [Crossref] [PubMed]