Molecular pathology for cholangiocarcinoma: a review of actionable genetic targets and their relevance to adjuvant & neoadjuvant therapy, staging, follow-up, and determination of minimal residual disease
Introduction
Cholangiocarcinoma (CCA) represents a group of epithelial cell tumors classified into intrahepatic CCA (iCCA) or extrahepatic based on anatomic location along the biliary tree (1). Extrahepatic CCA is further divided into perihilar (pCCA) and distal CCA (dCCA). The term biliary tract cancer (BTC) is a more inclusive term used to incorporate gallbladder cancer (GBC) into this group. These malignancies are relatively rare, and unfortunately, the majority of patients are diagnosed at later stages and with unresectable disease (2). Even in the small subset of patients who are candidates for curative-intent surgical resection, recurrence rates are high and 5-year survival rates are less than 30% (3,4). The current standard of care for patients with advanced stage CCA remains cisplatin plus gemcitabine based on the ABC-02 trial published in 2010, which improved the median overall survival (mOS) to just under 12 months compared to 8 months with gemcitabine alone (5). It is important to note, however, that although all are considered BTCs, each site of disease is unique in its presentation, surgical management, and molecular make-up. In recent years, molecular profiling of BTCs has revealed a wealth of potentially targetable genetic alterations, prompting numerous translational and clinical studies (6). The likelihood that a tumor will harbor a specific “actionable mutation” is unique to the site of disease. As demonstrated in other malignancies, personalized treatment strategies are instrumental in decreasing the burden of drug toxicity and improving patient outcomes (7,8). In this review, we illustrate the current status of targeted therapies by focusing on four examples, address their role in dictating staging and follow-up, and discuss potential applications to adjuvant and neoadjuvant therapy in order to push the field forward.
Discussion
Main actionable genetic targets
Here we present the current body of knowledge regarding the main molecular sites containing targetable genetic aberrations in BTC: fibroblast growth factor receptor (FGFR), isocitrate dehydrogenase 1 (IDH1), human epidermal growth factor receptor 2 (HER2), and microsatellite instability (MSI) (Figure 1). While this list is not comprehensive of all potential actionable mutations that have been uncovered in this malignancy, we chose these four to illustrate the overall status of this field.
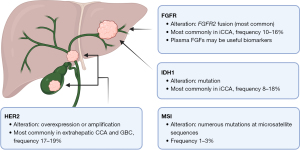
FGFR
Any FGFR genetic aberration is most commonly found in iCCA, and FGFR2 fusions, with over 50 different partners described, represent the vast majority (9,10). The earliest description of FGFR fusions in iCCA was published in 2013, based on two cases of FGFR2-BICC1 fusions (11). The incidence of FGFR2 fusion in iCCA ranges from 10–16%, although it has been reported up to 45% based on RNA sequencing (12,13) and as low as 5% based on the screening of patients for first-line clinical trials. Based on retrospective analysis, presence of an FGFR genetic aberration is associated with presentation at earlier stage, an indolent disease course, and longer overall survival (OS) (9). Thus, the presence of an FGFR2 fusion is believed to be a favorable prognostic biomarker, and given its association with earlier stage disease, its presence is believed to be more common in patients undergoing curative-intent resection.
Several phase II studies in locally advanced or metastatic CCA patients with FGFR alterations have demonstrated durable objective responses to an FGFR inhibitor, with an overall response rate (ORR) ranging from 14–42% and a median progression-free survival (mPFS) of 5.6–9 months (Table 1) (14-21). The largest of these studies were limited to patients with FGFR2 fusions or rearrangements. In the case of the FIGHT-202 trial of pemigatinib, results were stratified by patients with FGFR2 fusions or rearrangements versus any other FGFR mutation. While the former group had an ORR of 35.5% with a mPFS of 6.9 months, no patients in the latter group experienced a complete or partial response and the mPFS was only 2.1 months (19). Therefore, the presence of an FGFR2 fusion is currently considered the most “actionable” of the FGFR family. Food and Drug Administration (FDA) accelerated approval has since been granted for pemigatinib, infigratinib and futibatinib, pending confirmatory studies, for the treatment of patients with chemorefractory CCA that harbors an FGFR2 fusion or rearrangement.
Table 1
Molecular target | Trial identifier(s) | Phase | Treatment | Control arm | Genetic alteration included in selection criteria? | Number of patients eligible for treatment |
Median OS, mos. (95% CI) |
Median PFS, mos. (95% CI) |
ORR %, DCR % | No. of patients with response |
---|---|---|---|---|---|---|---|---|---|---|
FGFR | NCT02924376 (FIGHT-202) | II | Pemigatinib (INCB054828) | None | No | 146 (107 with FGFR2 rearrangement/fusion) |
21.1 (14.8–NR)^ |
6.9 (6.2–9.6) | 35.5, 82 |
3 CR, 35 PR |
NCT02150967 (–) | II | Infigratinib (BGJ398) | None | Any FGFR alteration | 122 (108 with FGFR2 rearrangement/fusion) |
12.2 (10.7–14.9) |
7.3 (5.6–7.6) | 23.1, 84.3 | 1 CR, 24 PR |
|
NCT02052778 (FOENIX-CCA2) | II | Futibatinib (TAS-120) | None | FGFR2 fusion/rearrangement | 103 | 20 (not reported) | 8.9 (not reported) | 41.7, 82.5 | –, – | |
NCT03230318 (FIDES-01) | II | Derazantinib (ARQ 087) | None | FGFR2 fusion | 103 | 15.5 (11.8–21.9) |
7.8 (5.5–8.2) | 21.4, 74.8 | 22 PR | |
NCT02699606* (LUC2001) | IIa | Erdafatinib (JNJ-42756493) | None | Any FGFR alteration | 22 | 40.2 (9.9–NR) |
5.6 (3.6–12.7) | 40.9, 81.8 | 1 CR, 8 PR |
|
NCT03656536 (FIGHT-302) | III | Pemigatinib | Gemcitabine/cisplatin | FGFR2 rearrangement | Study ongoing, no results have been published | |||||
NCT03773302 (PROOF 301) | III | Infigratinib | Gemcitabine/cisplatin | FGFR2 fusion/rearrangement | Study ongoing, no results have been published | |||||
NCT04093362 (FOENIX-CCA3) | III | Futibatinib | Gemcitabine/cisplatin | FGFR2 rearrangement | Study ongoing, no results have been published | |||||
IDH1 | NCT02989857 (ClarIDHy) | III | Ivosidenib | Placebo | IDH1 mutation | 187 (126 in treatment group) |
10.3 (7.8–12.4)# |
2.7 (1.6–4.2) | 2.4, 53.2 |
3 PR |
HER2 | NCT02091141* (MyPathway) | IIa | Pertuzumab + trastuzumab | None | HER2 overexpression or amplification | 39 | 10.9 (5.2–15.6) |
4.0 (1.8–5.7) | 23, 51 |
9 PR |
NCT04466891 (HERIZON-BTC-01) | II | Zanidatamab | None | HER2 amplification | Study ongoing, no results have been published | |||||
MSI | NCT02628067* (KEYNOTE-158) | II | Pembrolizumab | None | MSI-H/dMMR | 22 | 24.3 (6.5–NR) |
4.2 (2.1–NR) |
40.9, – | 2 CR, 7 PR |
NCT03875235 (TOPAZ-1) | III | Durvalumab + gemcitabine/cisplatin | Gemcitabine/cisplatin | No | 685 (451 in treatment group) |
12.8 (11.1–14.0)# |
7.2 (6.7–7.4) | 26.7, 85.3 | 7 CR, 84 PR |
*, these trials also included patients with other malignancies; only the CCA cohorts are reported here; ^, the results reported in this row are for the subset of patients with FGFR2 fusion; #, the results in these rows represent the treatment group. CCA, cholangiocarcinoma; OS, overall survival; CI, confidence interval; mos, months; PFS, progression-free survival; ORR, overall response rate; DCR, disease control rate; FGFR, fibroblast growth factor receptor; IDH1, isocitrate dehydrogenase 1; HER2, human epidermal growth factor receptor 2; MSI, microsatellite instability; MSI-H, microsatellite instability-high; dMMR, DNA mismatch repair deficiency; NR, not reached; CR, complete response; PR, partial response;
Post-hoc analysis of the FIGHT-202 trial revealed that patients who received second-line treatment with pemigatinib had longer mPFS and better response rates compared to patients who received second-line systemic therapy prior to trial enrollment, suggesting that earlier treatment is key for optimizing clinical efficacy (22). Three phase III trials are currently underway to compare futibatinib (FOENIX-CCA3, NCT04093362), infigratinib (PROOF 301, NCT03773302), and pemigatinib (FIGHT-302, NCT03656536) as first-line monotherapy against current standard of care gemcitabine/cisplatin. Enrollment in each of these trials has been challenging given the rarity of this disease, competing trials, and the lower observed incidence of an FGFR2 fusion compared to what was previously thought based on the phase II second-line studies.
Despite these promising results, primary and secondary resistance to FGFR inhibitors remains a concern. Resistance is thought to be influenced by co-occurring or acquired genetic alterations. For example, in the FIGHT-202 trial, a significantly shorter mPFS was seen in patients with a concomitant mutation in TP53, CDKN2A/B or PBRM1 (23). FOENIX-CCA2 showed a similar trend with TP53 co-mutation, although one patient did have a complete response (24). In four patients who progressed on either infigratinib or zoligratinib (debio-1347), new FGFR2 kinase domain mutations were found. Two of these patients achieved partial response and two had stable disease after treatment with futibatinib, highlighting the ability of this irreversible FGFR inhibitor to overcome such acquired resistance (25).
Currently, the presence of an FGFR genetic aberration has no impact on staging or follow-up recommendations. There is, however, preliminary evidence that plasma fibroblast growth factors (FGFs) may be useful biomarkers. In the FIDES-01 study of derazantinib, levels of FGF23 were increased on cycle 2 day 1, which was consistent with FGFR inhibition as previously published in other malignancies (26,27). A similar increase was seen in FGF19 and 21. These plasma biomarkers may serve as an adjunct to assessing response in addition to the typical methods of radiography and serum tumor markers.
IDH1
Similar to FGFR2 fusions, IDH1 mutations (mIDH1) are more commonly present in iCCA, with a reported average frequency of 8–18% (28). There is no clear association between mIDH1 and OS or time to progression, so it is not considered a prognostic biomarker. After promising results in a phase I study, the randomized, double-blind phase III trial ClarIDHy was designed to compare ivosidenib (AG-120), a small molecule inhibitor of mDH1, against placebo in patients with advanced CCA with mIDH1 who had progressed on previous therapy (NCT02989857). There was a significant improvement in mPFS (2.7 vs. 1.4 months, P<0.0001) and a favorable benefit in mOS (10.3 vs. 7.5 months, P=0.09) with the targeted therapy (Table 1) (29,30). After adjusting for crossover at the time of progression, the mOS for placebo was only 5.1 months, further emphasizing the improvement in OS seen with administering ivosidenib to those patients whose tumors harbored an mIDH1. In the last year, the FDA approved ivosidenib for the treatment of chemorefractory mIDH1 CCA. Interestingly, comprehensive genomic profiling of over 3,000 advanced iCCA revealed that there are significantly fewer co-occurring targetable genetic aberrations, as well as lower TMB, MSI-high, and PD-L1 expression, in mIDH (1 or 2) than in wild-type IDH (31).
HER2
HER2 overexpression and gene amplifications are found most commonly in extrahepatic CCA and GBC, at a rate of 17–19% (32). Although case reports suggest that patients with HER2 overexpression respond to HER2-targeting antibodies (33,34), initial phase I and II clinical trials employed broad inhibitors of EGFR and HER2 (lapatinib or afatinib) and failed to demonstrate any significant benefit (35-37). These trials were performed in an unselected group of patients, two of which later found no evidence of HER2 overexpression in any participant. There are currently two active phase II clinical trials in HER2− overexpressing BTCs, both of which employ HER2− specific antibodies (Table 1). Zanidatamab (ZW25) showed efficacy in a phase I trial with an ORR of 47% and is now being employed in a global phase IIb study (NCT04466891) (38). The MyPathway phase IIa basket trial employs a dual anti-HER2 regimen of pertuzumab and trastuzumab, and in the BTC cohort a 23% ORR was seen, with 9 of 39 patients achieving a partial response (NCT02091141) (39).
MSI/mismatch repair deficiency (MMR)
DNA mismatch repair deficiency (dMMR) leads to the accumulation of numerous mutations at repetitive (termed “microsatellite”) sequences, leading to high levels of MSI. The reported incidence of MSI in BTC has varied widely, largely due to the diversity of methods used for detection, but the true frequency is believed to be low: 1–3% in CCA and only 1.4% in GBC (40-42). A study in liver-fluke-related CCA in endemic Thailand, however, suggested an incidence up to 27% in iCCA and an association with poor survival (43). In cancers with dMMR and MSI, there is a known upregulation of checkpoint proteins such as programmed cell death ligand 1 (PD-L1) and cytokine T-lymphocyte-associated protein 4 (CTLA-4) (44,45) and an association with favorable response to checkpoint inhibition (46,47). The KEYNOTE-158 phase II trial enrolled patients with MSI-high/dMMR cancers who failed prior therapy and treated them with anti-programmed cell death protein 1 (anti-PD-1) antibody pembrolizumab. The CCA cohort had an ORR of 40.9%, with 2 patients exhibiting a complete response and 7 with a partial response (Table 1) (48). In the TOPAZ-1 phase III trial, an unselected group of patients received standard of care gemcitabine/cisplatin plus the PD-L1 inhibitor durvalumab or placebo (NCT03875235). The patients receiving durvalumab had an ORR of 26.7%, with a significant improvement in mOS to 12.8 vs. 11.5 months with placebo (Table 1) (49). PD-L1 expression was associated with better PFS but not OS in patients who received checkpoint blockade. MSI was high in 1.5% of patients, but over 50% of the patients in each group had an unknown status. While no associations with survival were able to be made based on the small sample size, the authors concluded that the low incidence of MSI-high tumors makes it unlikely to be the sole driver behind significant survival benefits seen with PD-L1 blockade. Repeat studies with comprehensive MSI data are needed, but currently the addition of durvalumab to gemcitabine/cisplatin is considered a standard of care approach for patients with advanced stage disease regardless of MSI status.
Adjuvant and neoadjuvant therapy
Similar to the ongoing evolution of the standard of care regimen for patients with unresectable CCA, the role of and optimal adjuvant therapy regimen for resected disease has yet to be defined (50). There is evidence that adjuvant chemoradiation, based on a single-arm phase II study (S0809), may positively impact local control in margin-positive resections and node-positive disease (51,52). The BILCAP trial recently published the results of 447 patients who underwent curative intent resection and were randomized to six months of oral capecitabine versus observation (53). The per-protocol analysis showed a significant improvement in mOS (53 vs. 36 months, P=0.028) and median recurrence-free survival (26 vs. 17 months, P=0.0093) in the capecitabine group compared to observation, while the intent-to-treat analysis did not meet statistical significance for the primary outcome of OS. Still, extrapolating from the ABC-02 data, gemcitabine/cisplatin is often utilized in the adjuvant setting. A phase III study (ACTICCA-1) is currently underway comparing gemcitabine/cisplatin to capecitabine (NCT02170090).
No studies to date have included genetic profiling as a basis for targeted adjuvant therapy. Further large-scale randomized trials in the adjuvant space that employ this personalized approach are warranted, but there are several factors which make this undertaking a challenge. Only about 20% of patients who present with CCA are candidates for resection (54), and actionable genetic alterations (as discussed in the previous section) are even rarer. Post-operatively, not all patients are candidates for or would be able to tolerate adjuvant therapy. If enrollment is limited to one particular mutation, the feasibility of such a study is threatened. Instead, we propose an umbrella study as a possible solution, in which the control arm receives capecitabine (per the BILCAP protocol) and the treatment arms receive targeted therapy based on the presence of an actionable genetic mutation. In this scenario, there would be multiple treatment arms based on the specific mutation or alteration that is present, such as FGFR2 fusion, mIDH1, HER2 amplification, MSI-high, etc. The feasibility, safety, and efficacy of combining targeted therapy with cytotoxic chemotherapy remains unknown at this time and is under investigation in the advanced disease setting.
Given the natural history of this disease with high recurrence rates after resection, optimizing preoperative therapy is the logical next step, much as we have done for esophageal, gastric, pancreas, and rectal cancers. Treatment in the neoadjuvant setting represents a completely underutilized space, as it harbors the potential to eradicate micrometastatic disease, downsize tumors, improve patient selection for resection, and ultimately improve survival. Furthermore, when treating in the neoadjuvant setting (as opposed to the adjuvant), there are opportunities to assess pathologic and radiologic response, as well as perform blood- and tissue-based correlative studies. The transition to the neoadjuvant setting needs to follow a stepwise approach, establishing feasibility and safety along the way, while considering the cytostatic versus cytotoxic nature of the drug. This transition can be accomplished by administering standard of care chemotherapy (i.e., gemcitabine/cisplatin) in the preoperative setting, which is being tested in EA2197 (OPT-IN) for incidentally-diagnosed GBC (NCT04559139). We can also employ a chemotherapy augmentation approach as was done in the NEO-GAP study where patients received preoperative therapy with gemcitabine/cisplatin/nab-paclitaxel prior to resection of oncologically high-risk iCCA (NCT03579771) (55). To push the field further and incorporate personalized targeted therapy in the neoadjuvant space, we have proposed an IRB -approved phase II study named OPT-IC (Optimal Preoperative Therapy for Intrahepatic Cholangiocarcinoma, NCT05514912), designed to assess the feasibility of administering neoadjuvant targeted therapy based on next-generation sequencing (NGS) performed on a preoperative core biopsy. While patients await their NGS results, both treatment arms will receive one cycle of nab-paclitaxel, gemcitabine and cisplatin. Patients bearing FGFR2 fusions or translocations will transition to receive an oral FGFR inhibitor for two cycles while those without an FGFR2 fusion will continue with chemotherapy for two more cycles. Patients with response, stable disease, or persistently resectable disease will then undergo surgery. We envision a myriad of further correlative studies that would be possible given collection of both pre- and post-treatment blood and tissue: from the identification of predictive factors for response, to examination of the effect that targeted therapy has at the cellular level. Future trials in the neoadjuvant space would likely incorporate an umbrella study design to include targeted therapies for other actionable mutations in multiple treatment arms as described for adjuvant therapy above. This personalized approach has the potential to improve the treatment strategy for patients with localized resectable disease.
Follow-up and minimal residual disease (MRD)
Tumor molecular profiling has yet to make an impact on patient follow-up or detection of MRD. Although not part of standard of care, one current option for assessing MRD is Guardant RevealTM, a blood test that detects circulating tumor DNA (ctDNA) based on genomic alterations and DNA methylation. Its use has demonstrated favorable sensitivity and specificity for identifying recurrence in colon and breast cancer (56,57). This method is “tumor-uninformed”, in that it detects a standardized panel of known genomic alterations. An alternative is SignateraTM, which detects ctDNA based on tumor whole-exome sequencing and is personalized for each patient. It has been shown to reliably predict recurrence in multiple cancer types (58-60). This “tumor-informed” approach, however, may be restricted when there is limited tissue or when tumor cellularity or DNA yield is low. In the phase II trial of infigratinib (BGJ398) in CCA, ctDNA was collected in three patients who experienced a short interval disease progression despite initial tumor regression (61). Analysis of ctDNA showed the presence of an FGFR2 V565F gatekeeper mutation at progression in all three patients, and additional FGFR2 kinase domain mutations in two patients. These findings represent ctDNA as a promising strategy for detecting therapeutic resistance in CCA, but its relevance to MRD remains largely unknown.
Conclusions
An increasing proportion of patients with biliary tract malignancies undergo molecular profiling at the time of diagnosis or at some point during their treatment sequence. There is an increasing body of evidence supporting that discrete genetic alterations in CCA are targetable with novel therapeutic agents and that such treatments offer significant clinical benefit for patients in the second-line setting. None of these genetic mutations yet dictate staging, follow-up or assessment of MRD and the future of their role in these settings is unclear. We stress that for patients with localized disease, focus should be turned to trials in the neoadjuvant setting, in addition to continued efforts in the first-line for unresectable disease. Ultimately, treating each patient with a personalized drug through targeted molecular therapy has the promise of improved outcomes with minimized side effects. Collaboration, at the national and international level, is crucial to success.
Acknowledgments
Funding: None.
Footnote
Provenance and Peer Review: This article was commissioned by the Guest Editors (Yuman Fong and David Kooby) for the series “Molecular, Protein, and Cellular Markers for HPB Cancers” published in Hepatobiliary Surgery and Nutrition. The article has undergone external peer review.
Conflicts of Interest: Both authors have completed the ICMJE uniform disclosure form (available at https://hbsn.amegroups.com/article/view/10.21037/hbsn-22-563/coif). The series “Molecular, Protein, and Cellular Markers for HPB Cancers” was commissioned by the editorial office without any funding or sponsorship. S.K.M. serves as the unpaid editorial board member of Hepatobiliary Surgery and Nutrition. The authors have no other conflicts of interest to declare.
Ethical Statement: The authors are accountable for all aspects of the work in ensuring that questions related to the accuracy or integrity of any part of the work are appropriately investigated and resolved.
Open Access Statement: This is an Open Access article distributed in accordance with the Creative Commons Attribution-NonCommercial-NoDerivs 4.0 International License (CC BY-NC-ND 4.0), which permits the non-commercial replication and distribution of the article with the strict proviso that no changes or edits are made and the original work is properly cited (including links to both the formal publication through the relevant DOI and the license). See: https://creativecommons.org/licenses/by-nc-nd/4.0/.
References
- Nakeeb A, Pitt HA, Sohn TA, et al. Cholangiocarcinoma. A spectrum of intrahepatic, perihilar, and distal tumors. Ann Surg 1996;224:463-73; discussion 473-5. [Crossref] [PubMed]
- de Groen PC, Gores GJ, LaRusso NF, et al. Biliary tract cancers. N Engl J Med 1999;341:1368-78. [Crossref] [PubMed]
- Khan SA, Davidson BR, Goldin R, et al. Guidelines for the diagnosis and treatment of cholangiocarcinoma: consensus document. Gut 2002;51:VI1-9. [Crossref] [PubMed]
- DeOliveira ML, Cunningham SC, Cameron JL, et al. Cholangiocarcinoma: thirty-one-year experience with 564 patients at a single institution. Ann Surg 2007;245:755-62. [Crossref] [PubMed]
- Valle J, Wasan H, Palmer DH, et al. Cisplatin plus gemcitabine versus gemcitabine for biliary tract cancer. N Engl J Med 2010;362:1273-81. [Crossref] [PubMed]
- Valle JW, Lamarca A, Goyal L, et al. New Horizons for Precision Medicine in Biliary Tract Cancers. Cancer Discov 2017;7:943-62. [Crossref] [PubMed]
- Pishvaian MJ, Blais EM, Brody JR, et al. Overall survival in patients with pancreatic cancer receiving matched therapies following molecular profiling: a retrospective analysis of the Know Your Tumor registry trial. Lancet Oncol 2020;21:508-18. [Crossref] [PubMed]
- Kittaneh M, Montero AJ, Glück S. Molecular profiling for breast cancer: a comprehensive review. Biomark Cancer 2013;5:61-70. [Crossref] [PubMed]
- Jain A, Borad MJ, Kelley RK, et al. Cholangiocarcinoma With FGFR Genetic Aberrations: A Unique Clinical Phenotype. JCO Precis Oncol 2018;2:1-12. [Crossref] [PubMed]
- Javle M, Bekaii-Saab T, Jain A, et al. Biliary cancer: Utility of next-generation sequencing for clinical management. Cancer 2016;122:3838-47. [Crossref] [PubMed]
- Wu YM, Su F, Kalyana-Sundaram S, et al. Identification of targetable FGFR gene fusions in diverse cancers. Cancer Discov 2013;3:636-47. [Crossref] [PubMed]
- Churi CR, Shroff R, Wang Y, et al. Mutation profiling in cholangiocarcinoma: prognostic and therapeutic implications. PLoS One 2014;9:e115383. [Crossref] [PubMed]
- Sia D, Losic B, Moeini A, et al. Massive parallel sequencing uncovers actionable FGFR2-PPHLN1 fusion and ARAF mutations in intrahepatic cholangiocarcinoma. Nat Commun 2015;6:6087. [Crossref] [PubMed]
- Voss MH, Hierro C, Heist RS, et al. A Phase I, Open-Label, Multicenter, Dose-escalation Study of the Oral Selective FGFR Inhibitor Debio 1347 in Patients with Advanced Solid Tumors Harboring FGFR Gene Alterations. Clin Cancer Res 2019;25:2699-707. [Crossref] [PubMed]
- Park JO, Feng YH, Chen YY, et al. Updated results of a phase IIa study to evaluate the clinical efficacy and safety of erdafitinib in Asian advanced cholangiocarcinoma (CCA) patients with FGFR alterations. J Clin Oncol 2019;37:4117.
- Javle M, Roychowdhury S, Kelley RK, et al. Infigratinib (BGJ398) in previously treated patients with advanced or metastatic cholangiocarcinoma with FGFR2 fusions or rearrangements: mature results from a multicentre, open-label, single-arm, phase 2 study. Lancet Gastroenterol Hepatol 2021;6:803-15.
- Droz Dit Busset M, Shaib WL, Mody K, et al. 47P Derazantinib for patients with intrahepatic cholangiocarcinoma harboring FGFR2 fusions/rearrangements: Primary results from the phase II study FIDES-01. Ann Oncol 2021;32:S376.
- Mazzaferro V, El-Rayes BF, Droz Dit Busset M, et al. Derazantinib (ARQ 087) in advanced or inoperable FGFR2 gene fusion-positive intrahepatic cholangiocarcinoma. Br J Cancer 2019;120:165-71. [Crossref] [PubMed]
- Abou-Alfa GK, Sahai V, Hollebecque A, et al. Pemigatinib for previously treated, locally advanced or metastatic cholangiocarcinoma: a multicentre, open-label, phase 2 study. Lancet Oncol 2020;21:671-84. [Crossref] [PubMed]
- Goyal L, Meric-Bernstam F, Hollebecque A, Morizane C, Valle JW, Karasic TB, et al. Updated results of the FOENIX-CCA2 trial: Efficacy and safety of futibatinib in intrahepatic cholangiocarcinoma (iCCA) harboring FGFR2 fusions/rearrangements. J Clin Oncol 2022;40:4009.
- Feng YH, Su WC, Oh DY, et al. Updated analysis with longer follow up of a phase 2a study evaluating erdafitinib in Asian patients (pts) with advanced cholangiocarcinoma (CCA) and fibroblast growth factor receptor (FGFR) alterations. J Clin Oncol 2022;40:430.
- Bibeau K, Féliz L, Lihou CF, et al. Progression-Free Survival in Patients With Cholangiocarcinoma With or Without FGF/FGFR Alterations: A FIGHT-202 Post Hoc Analysis of Prior Systemic Therapy Response. JCO Precis Oncol 2022;6:e2100414. [Crossref] [PubMed]
- Silverman IM, Hollebecque A, Friboulet L, et al. Clinicogenomic Analysis of FGFR2-Rearranged Cholangiocarcinoma Identifies Correlates of Response and Mechanisms of Resistance to Pemigatinib. Cancer Discov 2021;11:326-39. [Crossref] [PubMed]
- Bridgewater J, Meric-Bernstam F, Hollebecque A, Valle JW, Morizane C, Karasic T, et al. 54P Efficacy and safety of futibatinib in intrahepatic cholangiocarcinoma (iCCA) harboring FGFR2 fusions/other rearrangements: Subgroup analyses of a phase II study (FOENIX-CCA2). Ann Oncol 2020;31:S261-2.
- Goyal L, Shi L, Liu LY, et al. TAS-120 Overcomes Resistance to ATP-Competitive FGFR Inhibitors in Patients with FGFR2 Fusion-Positive Intrahepatic Cholangiocarcinoma. Cancer Discov 2019;9:1064-79. [Crossref] [PubMed]
- Kim KB, Chesney J, Robinson D, et al. Phase I/II and pharmacodynamic study of dovitinib (TKI258), an inhibitor of fibroblast growth factor receptors and VEGF receptors, in patients with advanced melanoma. Clin Cancer Res 2011;17:7451-61. [Crossref] [PubMed]
- Escudier B, Grünwald V, Ravaud A, et al. Phase II results of Dovitinib (TKI258) in patients with metastatic renal cell cancer. Clin Cancer Res 2014;20:3012-22. [Crossref] [PubMed]
- Boscoe AN, Rolland C, Kelley RK. Frequency and prognostic significance of isocitrate dehydrogenase 1 mutations in cholangiocarcinoma: a systematic literature review. J Gastrointest Oncol 2019;10:751-65. [Crossref] [PubMed]
- Zhu AX, Macarulla T, Javle MM, et al. Final Overall Survival Efficacy Results of Ivosidenib for Patients With Advanced Cholangiocarcinoma With IDH1 Mutation: The Phase 3 Randomized Clinical ClarIDHy Trial. JAMA Oncol 2021;7:1669-77. [Crossref] [PubMed]
- Abou-Alfa GK, Macarulla T, Javle MM, et al. Ivosidenib in IDH1-mutant, chemotherapy-refractory cholangiocarcinoma (ClarIDHy): a multicentre, randomised, double-blind, placebo-controlled, phase 3 study. Lancet Oncol 2020;21:796-807. [Crossref] [PubMed]
- Makawita S, Borad MJ, Carapeto F, et al. IDH1 and IDH2 Driven Intrahepatic Cholangiocarcinoma (IHCC): A comprehensive genomic and immune profiling study. J Clin Oncol 2021;39:4009.
- Galdy S, Lamarca A, McNamara MG, et al. HER2/HER3 pathway in biliary tract malignancies; systematic review and meta-analysis: a potential therapeutic target? Cancer Metastasis Rev 2017;36:141-57. [Crossref] [PubMed]
- Sorscher S. Marked radiographic response of a HER-2-overexpressing biliary cancer to trastuzumab. Cancer Manag Res 2013;9:1-3. [Crossref] [PubMed]
- May M, Raufi AG, Sadeghi S, et al. Prolonged Response to HER2-Directed Therapy in Three Patients with HER2-Amplified Metastatic Carcinoma of the Biliary System: Case Study and Review of the Literature. Oncologist 2021;26:640-6. [Crossref] [PubMed]
- Peck J, Wei L, Zalupski M, et al. HER2/neu may not be an interesting target in biliary cancers: results of an early phase II study with lapatinib. Oncology 2012;82:175-9. [Crossref] [PubMed]
- Ramanathan RK, Belani CP, Singh DA, et al. A phase II study of lapatinib in patients with advanced biliary tree and hepatocellular cancer. Cancer Chemother Pharmacol 2009;64:777-83. [Crossref] [PubMed]
- Moehler M, Maderer A, Ehrlich A, et al. Safety and efficacy of afatinib as add-on to standard therapy of gemcitabine/cisplatin in chemotherapy-naive patients with advanced biliary tract cancer: an open-label, phase I trial with an extensive biomarker program. BMC Cancer 2019;19:55. [Crossref] [PubMed]
- Meric-Bernstam F, Hanna DL, El-Khoueiry AB, et al. Zanidatamab (ZW25) in HER2-positive biliary tract cancers (BTCs): Results from a phase I study. J Clin Oncol 2021;39:299.
- Javle M, Borad MJ, Azad NS, et al. Pertuzumab and trastuzumab for HER2-positive, metastatic biliary tract cancer (MyPathway): a multicentre, open-label, phase 2a, multiple basket study. Lancet Oncol 2021;22:1290-300. [Crossref] [PubMed]
- Rashid A, Ueki T, Gao YT, et al. K-ras mutation, p53 overexpression, and microsatellite instability in biliary tract cancers: a population-based study in China. Clin Cancer Res 2002;8:3156-63.
- Goeppert B, Roessler S, Renner M, et al. Mismatch repair deficiency is a rare but putative therapeutically relevant finding in non-liver fluke associated cholangiocarcinoma. Br J Cancer 2019;120:109-14. [Crossref] [PubMed]
- Goeppert B, Roessler S, Renner M, et al. Low frequency of mismatch repair deficiency in gallbladder cancer. Diagn Pathol 2019;14:36. [Crossref] [PubMed]
- Limpaiboon T, Krissadarak K, Sripa B, et al. Microsatellite alterations in liver fluke related cholangiocarcinoma are associated with poor prognosis. Cancer Lett 2002;181:215-22. [Crossref] [PubMed]
- Howitt BE, Shukla SA, Sholl LM, et al. Association of Polymerase e-Mutated and Microsatellite-Instable Endometrial Cancers With Neoantigen Load, Number of Tumor-Infiltrating Lymphocytes, and Expression of PD-1 and PD-L1. JAMA Oncol 2015;1:1319-23. [Crossref] [PubMed]
- Llosa NJ, Cruise M, Tam A, et al. The vigorous immune microenvironment of microsatellite instable colon cancer is balanced by multiple counter-inhibitory checkpoints. Cancer Discov 2015;5:43-51. [Crossref] [PubMed]
- Le DT, Durham JN, Smith KN, et al. Mismatch repair deficiency predicts response of solid tumors to PD-1 blockade. Science 2017;357:409-13. [Crossref] [PubMed]
- Le DT, Uram JN, Wang H, et al. PD-1 Blockade in Tumors with Mismatch-Repair Deficiency. N Engl J Med 2015;372:2509-20. [Crossref] [PubMed]
- Marabelle A, Le DT, Ascierto PA, et al. Efficacy of Pembrolizumab in Patients With Noncolorectal High Microsatellite Instability/Mismatch Repair-Deficient Cancer: Results From the Phase II KEYNOTE-158 Study. J Clin Oncol 2020;38:1-10. [Crossref] [PubMed]
- Oh DY, Ruth He A, Qin S, et al. Durvalumab plus Gemcitabine and Cisplatin in Advanced Biliary Tract Cancer. NEJM Evid 2022; [Crossref]
- Shroff RT, Kennedy EB, Bachini M, et al. Adjuvant Therapy for Resected Biliary Tract Cancer: ASCO Clinical Practice Guideline. J Clin Oncol 2019;37:1015-27. [Crossref] [PubMed]
- Horgan AM, Amir E, Walter T, et al. Adjuvant therapy in the treatment of biliary tract cancer: a systematic review and meta-analysis. J Clin Oncol 2012;30:1934-40. [Crossref] [PubMed]
- Ben-Josef E, Guthrie KA, El-Khoueiry AB, et al. SWOG S0809: A Phase II Intergroup Trial of Adjuvant Capecitabine and Gemcitabine Followed by Radiotherapy and Concurrent Capecitabine in Extrahepatic Cholangiocarcinoma and Gallbladder Carcinoma. J Clin Oncol 2015;33:2617-22. [Crossref] [PubMed]
- Primrose JN, Fox RP, Palmer DH, et al. Capecitabine compared with observation in resected biliary tract cancer (BILCAP): a randomised, controlled, multicentre, phase 3 study. Lancet Oncol 2019;20:663-73. [Crossref] [PubMed]
- Bridgewater J, Galle PR, Khan SA, et al. Guidelines for the diagnosis and management of intrahepatic cholangiocarcinoma. J Hepatol 2014;60:1268-89. [Crossref] [PubMed]
- Maithel SK, Javle MM, Mahipal A, et al. NEO-GAP: A phase II single-arm prospective feasibility study of neoadjuvant gemcitabine/cisplatin/nab-paclitaxel for resectable high-risk intrahepatic cholangiocarcinoma. J Clin Oncol 2022;40:4097.
- Parikh AR, Van Seventer EE, Siravegna G, et al. Minimal Residual Disease Detection using a Plasma-only Circulating Tumor DNA Assay in Patients with Colorectal Cancer. Clin Cancer Res 2021;27:5586-94. [Crossref] [PubMed]
- Bidard FC, Hardy-Bessard AC, Bachelot T, et al. Abstract GS3-05: Fulvestrant-palbociclib vs continuing aromatase inhibitor-palbociclib upon detection of circulating ESR1 mutation in HR+ HER2- metastatic breast cancer patients: Results of PADA-1, a UCBG-GINECO randomized phase 3 trial. Cancer Res 2022;82:GS3-05.
- Reinert T, Henriksen TV, Christensen E, et al. Analysis of Plasma Cell-Free DNA by Ultradeep Sequencing in Patients With Stages I to III Colorectal Cancer. JAMA Oncol 2019;5:1124-31. [Crossref] [PubMed]
- Ococks E, Sharma S, Ng AWT, et al. Serial Circulating Tumor DNA Detection Using a Personalized, Tumor-Informed Assay in Esophageal Adenocarcinoma Patients Following Resection. Gastroenterology 2021;161:1705-1708.e2. [Crossref] [PubMed]
- Abbosh C, Birkbak NJ, Wilson GA, et al. Phylogenetic ctDNA analysis depicts early-stage lung cancer evolution. Nature 2017;545:446-51. [Crossref] [PubMed]
- Goyal L, Saha SK, Liu LY, et al. Polyclonal Secondary FGFR2 Mutations Drive Acquired Resistance to FGFR Inhibition in Patients with FGFR2 Fusion-Positive Cholangiocarcinoma. Cancer Discov 2017;7:252-63. [Crossref] [PubMed]