Pathologic assessment of hepatocellular carcinoma in the era of immunotherapy: a narrative review
Introduction
Hepatocellular carcinoma (HCC) is the most common and lethal disease worldwide (1,2). Due to the robust development in radiography and operative technique, about one-third of patients with HCC are able to receive curative therapy encompassing hepatic resection, liver transplantation, and ablation (3). However, there is still a huge therapeutic appeal of advanced HCCs unmet in the individualized therapy and precision medicine milieu. Indeed, the success of multi-target tyrosine kinase inhibitors such as sorafenib, lenvatinib, regorafenib, and cabozantinib unfolds a new era of targeted therapy for advanced HCC (4), nevertheless the dismal overall survival (OS) still urges constant exploration.
Within the past few years, immune checkpoint inhibitor (ICI)-related therapy has gradually exhibited as an available method for a series of cancer types (5). Among sophisticated immune checkpoint network, the programmed cell death 1 (PD-1) and PD-1 ligand 1 (PD-L1) axis, as well as cytotoxic T lymphocyte antigen 4 (CTLA4) are the pivotal backbones of immunotherapeutic pharmacological design at present (6). PD-L1 may be upwards on tumor cells, stromal cells, and myeloid cells and suppresses immune feedback by binding with its two receptors, PD-1 and CD80 on T cells or antigen presenting cells (7). The mutual effect of PD-L1 and PD-1/CD80 inhibits T cell proliferation, cytokine production, and cytolytic activity bringing about functional inactivation or exhaustion of T cells (8). CTLA-4 constitutively expresses on regulatory T (Treg) cells and up-regulates on activated T cells (9). When CTLA-4 binds to its ligands CD80 and CD86 on antigen presenting cells, T cell activation will be attenuated thereafter (10). The above immune checkpoint molecules physiologically maintain homeostasis and prevent autoimmune diseases (11), yet HCC and other cancers also express them on tumor cells and stromal cells in the tumor microenvironment (TME) so as to utilize these mechanisms to ignore and evade anti-tumor immune effect (12,13). A succession of ICIs are tailored to break up this abnormal immune phenomenon and liberate the functional immune cells to attack tumor (14).
As noted in Table 1, ICI is developing rapidly with dramatic potential in the treatment for advanced stage HCC (15). Atezolizumab, durvalumab, pembrolizumab, nivolumab, ipilimumab, dostarlimab-gxly have been ratified by the National Comprehensive Cancer Network (NCCN) as the first-line or subsequent-line therapy options of advanced HCC in the form of single agent or drug combination (16). In this scenario, it is reasonable to explore expanding the indication of ICIs in the management of HCC in other stages. There have been some pilot studies to apply ICIs to the neoadjuvant, conversion, and adjuvant therapy of HCC and some of which have acquired positive results (17,18). Predictably, immune therapy is potential to penetrate into the whole-course surveillance of HCC patients in the near future.
Table 1
Immune checkpoints | ICIs |
---|---|
PD-1 | Nivolumab |
Pembrolizumab | |
Tislelizumab | |
Camrelizumab | |
Toripalimab | |
Sintilimab | |
PD-L1 | Durvalumab |
Atezolizumab | |
CTLA-4 | Tremelimumab |
Ipilimumab |
ICI, immune checkpoint inhibitor; HCC, hepatocellular carcinoma; PD-1, programmed cell death 1; PD-L1, PD-1 ligand 1; CTLA-4, cytotoxic T lymphocyte antigen 4.
As for pathology, two issues are of paramount importance in the setting of HCC with immune therapy. First, although the Response Evaluation Criteria in Solid Tumors (RECIST) is still the gold standard to measure the response of oncotherapy (19), many studies showed an ambiguous correlation between the prognostic value provided by new agents and the evaluating results by this standard in HCC (20,21). Hence it is urgent to establish a feasible strategy to justify appraise and quantify the immunotherapeutic-related pathologic response (PR) of the resected HCC specimens that underwent neoadjuvant/conversion ICI-related therapy. Second, in considering that only a subset of HCC patients derives clinical benefit from ICI-related therapy, it is important to discover some reliable pathologic biomarkers to synergistically predict the treatment efficiency so as to optimize the stratified precise adjuvant or therapeutic immunotherapy (22). Focusing on these two concerns, in this review, we aim to summarize the involved studies and propose our viewpoints on the outstanding questions. We present this article in accordance with the Narrative Review reporting checklist (available at https://hbsn.amegroups.com/article/view/10.21037/hbsn-22-527/rc).
Methods
We searched PubMed for the following keywords: hepatocellular carcinoma, immunotherapy, immune checkpoint inhibitor, immune checkpoint blockade, conversion therapy, neoadjuvant therapy, adjuvant therapy, biomarker, pathologic evaluation, pathologic assessment. Only the published articles till February 2023 in PubMed database were included and no limit was put on the study design type.
Histopathologic assessment of specimens with ICI therapy
There have been some controversial high-level evidences discussing whether preoperative neoadjuvant treatment could benefit surgical prognosis of HCC (23,24). An orchestrated immunotherapeutic protocol is potential to direct this argument in a positive orientation (25,26). For instance, a phase 1b study devoted to the feasibility of neoadjuvant nivolumab and cabozantinib in patients with unresectable HCC with 80% of patients achieving curative resection, and 42% of patients obtaining major pathologic response (MPR) (27). Another single-arm phase 2 trial enrolled 21 patients with resectable HCC and gave neoadjuvant cemiplimab with 20 patients underwent surgical resection afterwards, among whom significant or partial tumor necrosis was observed in seven cases and the remaining patients maintained stable disease (28). One more study published in the same period evaluated the safety and tolerability of perioperative nivolumab plus or not plus ipilimumab in resectable HCC with 6 of 27 patients achieving MPR (29). A recent clinical trial explored the efficacy and safety of neoadjuvant camrelizumab plus apatinib in patients with resectable HCC and objective response rate was reached in 33.3% of patients based on modified RECIST (30). A retrospective study from China also validated that about 15.9% of patients with unresectable HCC underwent successful conversion with subsequent radical resection because of the initial combination therapy with anti-PD-1 antibodies and tyrosine kinase inhibitors (31). The aforementioned studies powerfully prove the clinical value of immunotherapy on the neoadjuvant and conversion therapy of HCC and promote the relevant clinical practice (Table 2). With the sharp increase of HCC specimens underwent immunotherapy, how to conduct a standardized pathologic assessment to better prompt the treatment effect and prognosis has become a momentous concern for pathologists.
Table 2
Agents | Number of patients | Number of resections | Number of MPR | Number of PCR | Reference |
---|---|---|---|---|---|
Nivolumab + cabozantinib | 15 | 12 | 5 | 1 | (27) |
Cemiplimab | 21 | 20 | 4 | 3 | (28) |
Nivolumab alone or + ipilimumab | 27 | 20 | 6 | 5 | (29) |
Camrelizumab + apatinib | 20 | 17 | 4 | 1 | (30) |
PD-1 blockade + tyrosine kinase inhibitor | 63 | 10 | Not available | 6 | (31) |
ICI, immune checkpoint inhibitor; HCC, hepatocellular carcinoma; PD-1, programmed cell death 1; MPR, major pathologic response; PCR, pathologic complete response.
Sampling method
Normalized sampling is the premise of any consistent pathologic evaluation. However, it should be of great concern that a large number of articles on pathologic measurement of various cancer types after neoadjuvant therapy avoid this crucial prerequisite. Furthermore, to our knowledge, none of the clinical practice guidelines compiled by the European Association for the Study of the Liver (32), the American Association for the Study of Liver Diseases (33), and the NCCN (16), as well as the standardizing pathology reporting formulated by the International Collaboration on Cancer Reporting (34) and the College of American Pathologists (35), mentioned any sampling schemes of HCC. By comparison, the seven-point sampling protocol first suggested by us has been widely used on the HCC specimens in China (36,37). Indeed, we recognize that this sampling method is mainly delivered in the initial untreated HCC with simultaneously juggling the workload and clarifying the diagnosis. It may be insufficient to accurately evaluate the PR of resected HCC with neoadjuvant/conversion therapy (38).
Added tissue blocks should be submitted from complete resected HCC specimens with preoperative therapy, but it is essential to exactly on behalf of the tumor bed and can be retrospectively mapped to the gross and/or radiologic examination (39). Unfortunately, the appropriate sampling extent is still vacant which is largely owing to the tardy research progress of preoperative therapy of HCC. Herein, we would like to introduce some referential sampling methods used in other cancer types (Table 3). First, the proposal of the pathologic assessment of residual disease in the specimens of breast cancer after neoadjuvant therapy raised by the Residual Disease Characterization Working Group of the Breast International Group-North American Breast Cancer Group deserves attention, in which recommended that the tissue blocks reflecting the full face of the pretreatment area of involvement should be taken of every 1 cm slice containing pretreatment area of involvement or five representative blocks of a cross-section of pretreatment area of involvement per 1–2 cm of pretreatment size, up to a total maximum of about 25 blocks for very large tumors (40,41). This method also has been applied to the neoadjuvant anti-PD-1 specimens of resected non-small cell lung carcinoma and developed an immune-related PR criterion (42). Moreover, for the neoadjuvant specimens of resected lung cancer, the International Association for the Study of Lung Cancer paid more attention to the section of the maximum dimension of the tumor bed and blocks should be gathered from the entire cross-section of the tumor bed to structure complete histologic sectioning (43). For the evaluated approach of treated pancreatic ductal adenocarcinoma, Pancreatobiliary Pathology Society rendered that small tumors (≤2 cm) should be entirely submitted and large tumors (>2 cm) might be sampled generously with representative sections (at lowest two sections per centimeter of the tumor should be sampled) (44). The specimen handling of pan-tumor pathologic evaluation to PD-1/PD-L1 blockades designed by Stein et al. was that one complete cross-section from the maximum dimension of the tumor bed should be collected and cut into slides, with an extra one section per centimeter taken for the leftover specimen. For tumors with large dimension (>5 cm), submitting every-other section or even every third section across the largest cross-sectional diameter is rational (45). Other various sampling methods suiting for gastrointestinal tumors (47) and melanoma (48) that underwent neoadjuvant therapy are not listed because of significantly different growth pattern from HCC. The similarity of aforesaid sampling protocols is that: sufficient sampling of the maximum cross-section of the tumor bed and widespread sampling of other cross-sections of the tumor bed. The former section is most likely to contain the most aggressive cell population of the tumor which represents the most malignant biological behavior and the most powerful drug resistance of the tumor. The latter section includes the main tumor cells which are on behalf of the subjective therapeutic response. However, although these sampling schemes are pragmatic for pathological practice, there still exists a wide gap from comprehensive assessment.
Table 3
Cancer type | Protocol | References |
---|---|---|
Liver cancer | Seven-point sampling method: 4 tissue blocks are sampled from the margin between the tumor and tumor-adjacent liver tissue and 1 tissue block is sampled from tumor core | (31,36,37) |
Breast/lung cancer | Tissue blocks reflecting the full face of the pretreatment area of involvement are taken of every 1 cm slice containing pretreatment area of involvement or 5 representative blocks of a cross-section of pretreatment area of involvement per 1 to 2 cm of pretreatment size, up to a total maximum of about 25 blocks for very large tumors | (40-42) |
Lung cancer | Tissue blocks are gathered from the entire cross-section of the maximum dimension of tumor bed to structure complete histologic sectioning | (43) |
Pancreatic cancer | Small tumors (≤2 cm) are entirely submitted and large tumors (>2 cm) are sampled with representative sections (at lowest two sections per centimeter of the tumor are sampled) | (44) |
Solid tumor | One complete cross-section from the maximum dimension of the tumor bed is collected and cut into slides, with an extra one section per centimeter taken for the leftover specimen or submitting every-other section or even every third section across the largest cross-sectional diameter is rational for tumors with large dimension (>5 cm) | (45) |
Breast cancer | A minimum of one block per centimeter of pretreatment tumor size or at least 10 blocks in total with 3 to 5 mm slices is prepared, whichever is greater | (46) |
ICI, immune checkpoint inhibitor.
There is no perfect and universal sampling scheme for assessment of HCC with preoperative immunotherapy up to now, on the other hand, we believe that the underlying purpose of this evaluation is to clarify the efficacy and prompt the prognosis. Overemphasizing the comprehensiveness of sampling may be unrealistic for HCC with complex lesions, such as huge volume or multiple nodules (38). The guidance document Pathological Complete Response in Neoadjuvant Treatment of High-Risk Early-Stage Breast Cancer: Use as an Endpoint to Support Accelerated Approval released by the US Food and Drug Administration stated that specimens from such patients should have a minimum of one block prepared per centimeter of pretreatment tumor size, or at least 10 blocks in total, whichever is greater, with 3 to 5 mm slices (46). We tend to the standpoint that a consistent number of sampling blocks rather than submission of entire tumor may be more suitable for achieving comparability among medical centers and is conformed to the actual work. Our study also revealed that the area proportion of tumor necrosis after preoperative transcatheter arterial chemoembolization (TACE) was significantly associated with the early recurrence of HCC with the pathologic evaluation based on the seven-point sampling method (49).
Immunotherapy-related histopathologic response
When immune checkpoint molecules on tumor cells are aberrantly activated, the host immune system will be suppressed thereby, ICIs can subvert this established cacoethic condition, concurrently if this reversion is too intense, it is unsurprising that a hyperactivation of the immune cells will be triggered with consequent immune-related adverse events (50,51). Given this premise, both the histological performance of tumor and liver should be included in the morphological observation of immune-related histopathologic response.
For the parenchyma of tumor, the architectural pattern of the viable residual tumor should be regarded. Two previous studies suggested that the majority of HCC followed by loco-regional ablation and TACE could be subdivided into three types: multiple nodules separated by fibrous septa, single nodule, and single nodule with smaller adjacent satellite nodules (Figure 1A-1C) (52,53). Although this classification has no prognostic value in patients of HCC with liver transplantation, we suppose that the distribution of remaining tumor cells may indicate the response to ICI treatment and possible recurrence patterns as there have been some studies confirming that the growth pattern was a significant prognostic factor of HCCs with liver resection (54,55). It is explicable that a mass composed of multiple nodules separated by fibrous septa may be more difficult to render necrosis because the partition effect of fibrous tissue across the tumor may assist tumor cells in escaping from destruction of ICIs. Single nodule with smaller adjacent satellite nodules may point out the condition of tumor spread within intrahepatic vessels and predispose to early recurrence. Besides, partial tumor cells of HCC underwent ICI treatment show the characteristic of degeneration, including tumor cells shrunken with nuclear pyknosis and cytoplasmic acidophilic change or tumor cells enlarged with malformed vacuolar nuclei and prominent nucleoli (Figure 2A,2B), which is sometimes confusing with tumor cells with poorly differentiated. Ki-67 stain (negative vs. positive) and distribution of tumor cells (scattered vs. aggregated) are useful in differentiated diagnosis (56). The transformation of immunophenotype of tumor cells is not unexpected and the positive stain of cholangiocyte marker, such as Cytokeratin 19, may signal drug resistance of tumor cells (57).
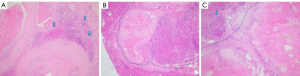
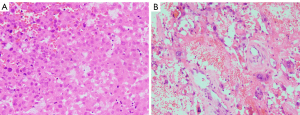
Tumor necrosis was considered as a parameter associated with clinical outcome of various cancer types with neoadjuvant therapy (58-60). However, the collaboration between the necrosis pattern and treatment efficacy of ICIs is unclear yet in HCC. Intriguingly, a retrospective study compared the prognosis between HCC patients showing coagulative necrosis and liquefactive necrosis after TACE based on computed tomography and concluded that HCC with liquefactive necrosis was preferred to have a worse prognosis (61). Another research found that HCC patients who received TACE combined with molecular targeted agents plus ICIs had more liquefactive tumor necrosis than patients who received TACE combined with molecular targeted agents (62). Different necrotic pattern may depend on the coordination the formation of tumor tissue necrosis and its absorption. Generally, the tumor necrotic tissue is dissolved and liquefaction by the action hydrolase released by neutrophil or engulfed by the macrophages, and then both of them were removed by the peripheral veins and lymphatics (62). Based on the above results, the ICIs enhance the anti-cancer effect, but also result in increased liquefactive necrosis formation, which may represent adverse outcome. Thus, more studies should be conducted to investigate the optimal dose of ICIs in clinical practice. The necrosis pattern of HCC after ICI therapy appears including coagulative necrosis with/without retaining the tumor structure and liquefactive necrosis with neutrophilic infiltration (Figure 3A-3C), which may embody the different effects of ICIs. We recommend distinguishing and reflecting in the pathologic report.
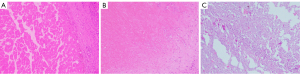
The characteristics of intratumoral stroma mainly reflect the TME of HCC (63). Recent advances in preclinical and clinical studies have increasingly defined the role of TME in the tumorigenesis and progression of HCC and facilitated the development of novel molecular targets (64). Fibrosis with infiltrated inflammatory cells, deposited hemosiderin, reactive ductular, hemorrhage, as well as cholesterol cleft are frequently observed in the HCC specimens underwent ICI therapy (Figure 4A-4D). Generally, these stromal changes may be either as intrinsic characteristics of tumor or secondary changes as a response of ICI therapy, but no specific method has been recognized to discriminate the causation of these stromal phenomena. Remarkably, continuous researches confirmed dramatic prognostic value of stromal component in the immune therapy (65), which reminds us to establish a relevant quantitative assessment standard (details below).
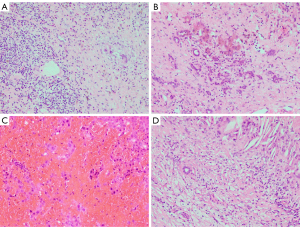
Patients treated with PD-1/PD-L1 blockades and anti-CTLA-4 antibodies were associated with 5–10% and up to 15% risk of hepatic injury, respectively (66). What is more, the incidence rate of hepatotoxicity soared to 30% in tumor patients who received a combination therapy of anti-CTLA-4 and anti-PD-1/PD-L1 inhibitors (67-69). Accordingly, it is equally essential to clarify the characteristics of these ICI-induced liver injuries in the pathologic report. To date, the morphologic changes of liver affected by ICIs are well described but incongruent. For instance, De Martin et al. (70) detected that hepatotoxicity-related histomorphology corresponding to anti-CTLA-4 antibody and anti-PD-1/PD-L1 antibody were granulomatous hepatitis (including fibrin ring granulomas and central vein endotheliitis) accompanied with remarkable CD8+ lymphocytes as well as lobular hepatitis accompanied with proportional CD4+ and CD8+ lymphocytes, respectively. Zen et al. (71) found that patients treated with ipilimumab or nivolumab showed predominant lobular hepatitis and mild portal inflammation with presence of large numbers of CD3+ and CD8+ lymphocytes plus small amounts of CD20+ B cells and CD4+ T cells. Johncilla and co-authors (72) concluded that the morphologic characteristic of hepatic injury observed in the majority of patients with ipilimumab was an active panlobular or zone 3 hepatitis with marked CD8+ T cells, admixed histiocytes, scattered plasma cells, and eosinophils. Simoes and his colleagues (73) approved that bile duct damage and prominent ductular reaction rather than perivascular changes and hepatitis were the main patterns of non-tumor liver of HCC underwent nivolumab therapy. To sum up, the manifestations of ICI-induced liver injury reported in the literatures are no known pathognomonic findings (74). We supposed that these heterogenetic results are owing to three reasons. First, as the majority of patients of HCC had underlying viral hepatitis, it is hard to evaluate the pertinent hepatic changes related to ICI therapy. Second, different morphologic performances may represent short-term or long-term responses to immunotherapy under the influence of immune cells with impaired cellular functions. Third, varied immune checkpoint molecules differentially enrich in various targeted cells of liver so as to raise diverse tissue damage. It is necessary for pathologists to routinely report the pathologic changes in non-tumor liver, such as bile duct reaction, vascular/perivascular inflammation, granuloma formation, as well as the grading of hepatitis for HCC patients with ICI-related therapy. The ultimate goals of the diagnosis of liver injury are to differentiate background liver disease, interpret hepatotoxicity, and optimize patient management.
Quantitative pathologic response rate
Pathologic complete response (PCR) and MPR have been regarded as the surrogate indicators of tumor response to neoadjuvant/conversion therapy in order to expedite assessment of the effectiveness of the agent being tested in clinical trials (75-77). From the pathological perspective, there is a lack of sound, standardized, reproducible algorithm to measure the PR rate of HCC with no consensus or guideline discussing this issue. The confused rules of various tumor regression grading systems resulting in chaotic assessment (78). We advocate the metering method by using percentage to record the PR rate of HCC after ICI therapy. After gaining initial experience, an algorithm of tumor regression grading system will be designed verifiably pointing at HCC with ICI therapy. The following controversies and challenges in the assessment of PR rate are highlighted and waiting for overcome.
What needs to be clarified firstly is that the absolute sense of PCR is delimited as no viable tumor cells after entirely sampling and slicing all specimens, which is almost impossible to be carried out in the real world, yet countless studies used this concept. In this regard, we recommend expounding the scope of sampling before using this terminology. Second, MPR has been authenticated as a valuable signal of great prognosis of tumor with neoadjuvant therapy (79). An optimal threshold of MPR ought to be orchestrated for efficient evaluation of ICI therapy and prognostic forecast. The cut-off value of ≤10% residual viable tumor was frequently used to define MPR and widespread conducted in various cancer types (77,80,81). Chinese standardization for the diagnosis and treatment of HCC likewise adopted this critical value to delimit MPR (82). However, the data-driven studies and rigorous statistical analysis rather than the current anecdotal approach are indispensable for addressing the best cut-off value of MPR. Third, it is intelligible that a wider range of necrosis is largely parallel with a superior curative efficacy of ICIs, but for the HCC with incomplete PR, dichotomous implications of tumor necrosis and residual tumor should be taken into account: (I) treatment response for the ICIs; (II) filtered drug-resistance tumor cells. The latter one may lead to more invasive tumor recurrence/metastasis. There has been no literature considering the significance of the different morphological characteristics of residual tumor cells in the pathologic assessment. A risk scoring calculator for the viable HCC cells with diverse histological grading, Ki-67 positive rate, tumor distribution, etc. may be required for further precisely appraising the PR of HCCs with accordant tumor necrosis rate. Fourth, it is still questionable whether the regions of fibrotic, hemorrhage, and other stromal changes should be brought into the area of tumor bed (38). Different approaches are conducted in the same cancer type causing a wide variation of results. We suggest that the whole area within the tumor margin should be deemed as the tumor bed as HCC is a kind of malignant tumor with expansive growth pattern generally forming a well-circumscribed capsule. Furthermore, residual microvascular invasion and satellite lesion/foci in adjacent liver is better to be reported independently because of their invasiveness. Fifth, significant interobserver variation still persists, especially for HCC with multiple nodules, dispersive growing pattern, cystic degeneration, and massive fibrosis, despite best efforts to minimize subjectivity. Combined with large-section histopathology (83) and artificial intelligence pathology (84,85) is a promising means to solve this issue in the future.
Potential predicted biomarkers of treatment response of ICI
Despite the extraordinary advancements in immunotherapy, the majority of patients with advanced HCC receiving ICIs do not derive survival benefits. The response rates of ICI monotherapy for HCC range from 15–23% and elevate to approximately 30% after combination treatment (86). Moreover, witnessing the extremely high postoperative recurrence rate of HCC, the clinicians naturally attempt to deliver ICIs into the postoperative adjuvant treatment of HCC as a synergism on prognostic improvement. As such, constant researches are promoting in deciphering predictive biomarkers of ICI treatment response to enable precise medicine, defeat drug resistance, reduce side effects and minimize medical cost in HCC immunotherapy. Unsatisfactory, in contrast with the significant progress in non-small cell lung cancer and melanoma (87), the selected biomarkers predicting ICI response almost have been verified with poor sensitivity and specificity in HCC (88). The marked intratumoral heterogeneity of HCC is supposed to leave this predicament (89). As a previous study revealed that the heterogeneity of tumor components was detectable in 87% of cases of HCC in which only morphology, several immunohistochemical markers, and individual molecular variations were taken into account (90). Furthermore, the deep sequencing of genome impelled the cognition that heterogeneity of HCC existed not only in the tumor cells but in the infiltrated immune cells (91). Owing to these complex tumor-immune heterogeneities, it is comprehensible that single biomarker may be insufficient to qualify for predicting immunotherapy response of HCC. We conjecture that combined application of biomarkers and testing multisite of tumor is hopeful to settle this issue but still need more evidence. In this part, we aim to summarize some salient and practical pathologic biomarkers that are prospective in the prediction of ICI response to HCC (Table 4).
Table 4
Factors | Potential association with immunotherapy | Possible assay type for biomarker assessment |
---|---|---|
TIL | Positive or negative | Histopathology, immunohistochemistry |
Intratumoral TLS | Positive | Histopathology, immunohistochemistry |
PD-L1 expression | Positive | Immunohistochemistry |
Wnt/β-catenin mutation | Negative | NGS, immunohistochemistry |
MSI and MMR | Positive | NGS, polymerase chain reaction, immunohistochemistry |
TMB and tumor neoantigen | Positive | NGS |
JAK-1/2 mutation | Negative | NGS |
PTEN-STAT3 mutation | Negative | NGS |
TGF-β expression | Negative | NGS |
TIL, tumor-infiltrating lymphocyte; TLS, tertiary lymphoid structure; PD-L1, programmed cell death 1 ligand 1; MSI, microsatellite instability; MMR, mismatch repair; TMB, tumor mutational burden; JAK, Janus tyrosine kinases; PTEN, phosphatase and tensin homologue; STAT3, signal transducer and activator of transcription 3; TGF-β, transforming growth factor beta; NGS, next-generation sequencing.
Tumor-infiltrating lymphocyte (TIL)
The immune cells in the TME are considered as the main responders of ICI (92). The TME of HCC consists of different populations of immune cells, which mainly include TIL, tumor-associated macrophage, tumor-associated neutrophil, and myeloid-derived suppressor cell (64). More in-depth clinical studies were conducted in the TIL along with wider application into medical procedures.
TIL is on behalf of the host’s anti-tumor response and is regarded as the central immune component (64). TIL affects hepatocarcinogenesis not only across directly manipulating the adaptive immune system and cytokine interactions, but also via modulating the innate immune system and angiogenesis (93,94). A stepwise descent in survival time has been confirmed in HCC with high-, intermediate- and low-density of TILs (95). Among the diverse subsets of TIL, CD8+ T cells and Foxp3+ Treg cells play the crucial roles in the activation and suppression of anti-tumor immunoreaction, respectively. In addition, CD3+ T cells and CD4+ T cells are also indispensable members of TIL while their prognostic value and influence on immunotherapy of HCC is more obscure and debatable, hence a more detailed discussion is not attempted (96). Activated CD8+ T cells can destroy tumor cells by releasing granzymes and perforin and then inducing apoptosis mediated by the death ligand/receptor. They can also regulate the immune system against tumor cells by secreting cytokines (97). Foxp3+ Treg cells accelerate tumor proliferation through suppressing anti-tumor immune response and simultaneously advance tumor progression by controlling tumor-related inflammation and sustaining peripheral tolerance (98). For HCC, several investigations have stated that the high density of CD8+ T cells in the invasive margin, intratumoral, and perivessel region all had a positive relationship with prognosis (99,100). Conversely, HCCs with higher density of Foxp3+ Treg cells had worse survival (101). Fueled by the close link between TIL and immunotherapy, available evidence suggests its predictive ability in the ICI-related therapy of HCC (102). For instance, there was a trend significance between worse clinical outcome in patients of HCC with nivolumab monotherapy and lower TILs of CD3+/CD8+ cells (103). Among patients with HCC receiving paired biopsies before and after tremelimumab monotherapy, those without tumor remission had lower TILs of CD3+ and CD8+ cells than responders (104). For the patients treated with atezolizumab and bevacizumab, pre-existing high intratumoral CD8 T cell density and strong expression of PD-L1 were associated with better prognosis with therapy, and poorer clinical benefit was associated with high Treg to effector T cell ratio (105,106). The ratio of PD-1+ CD8+ T cells and PD-1+ Treg cells in the TME also could forecast the efficacy of PD-1 blockades (107). HCC with a discrete population of PD-1 high CD8+ T cells was perhaps more susceptible to ICI-related therapy (108). High expression of Tregs and of Foxp3 showed a negative correlation with the expression of a 11-gene signature comprised genes involved in interferon-γ signaling, antigen presentation, and chemotaxis, which represented a resistant response to anti-PD-1 therapy (109,110).
From the perspective of routine pathology, a standardized method to assess the density, type, and distribution of various immune cells in the TME of HCC is still without framework. The methodologies of evaluation of TIL proposed by the International TILs Working Group (111) and the International Immuno-Oncology Biomarkers Working Group (112) are notable. Both consensuses consented that TIL was composed of the stromal compartment (stromal TIL) and the tumor cell compartment (intratumoral TIL). Intratumoral TIL is defined as lymphocytes that have immediate contact with tumor cells without intervening stroma, while stromal TIL is scattered located in the stroma between the tumor nests without directly interacting with tumor cells. An assessment of average TILs in the whole tumor area rather than hotspot area was recommended with a magnification of ×200–400. The discernible difference between these two tutorials was whether the intratumoral TIL should be separately reported. Our experience is that the intratumoral TIL in HCC just as breast cancer is an irreproducible evaluation parameter among pathologists, which is generally present in fewer cases and are laborious to observe on H&E-stained slides than stromal TIL. Therefore, in our opinion, exclusive of the research purpose, a formal report on the stromal TIL is satisfactory for reference of ICI treatment. The denominator used to calculate the stromal TILs is the area of stromal tissue, rather than the number of stromal cells (the percentage of mononuclear inflammatory cells in the total intratumoral stroma). No threshold of TIL for a clinically relevant has been acknowledged in HCC so far. A continuous variable of TIL is more appropriate to be informed in pathologic report nowadays in order for further exploration. Moreover, a panel of immunohistochemical markers, such as CD3, CD4, CD8, CD25, Foxp3, CD20, CD38, CD138, CD68, and CD163, can assist in distinguishing various immune cells, and depicting their spatial distribution.
Intratumoral tertiary lymphoid structure (TLS)
TLS is the organized aggregate of immune cells that form in nonlymphoid tissues which are highly analogue to secondary lymphoid organs anatomically (Figure 5A-5C) (113). TLS consists of T cell and B cell components, differentiated high-endothelial veins, and follicular dendritic cell networks supporting the germinal center reaction (114). As a specific conformation of immune cells, TLS is involved in a series of immune processes, namely, T cell priming and polarization, as well as B cell activation, differentiation, and secretion of antibodies (113). For HCC, a study showed the presence of intratumoral TLS, especially mature TLS, strongly indicated a great prognosis. Concurrently, HCC tumor with TLS was more frequent with PD-1 high expression (115). The aforementioned results can accordingly be speculated that TLS may be one of the decisive sites of action for activated immune cells that boost the anti-tumor immune responses. Recently, an interesting clinical trial performed by Ho and his colleagues showed that a great PR of HCC patients who underwent neoadjuvant cabozantinib and nivolumab was significantly correlated with the presence of intratumoral TLS (27). In view of this, it is plausible that intratumoral TLS may serve as a marker of ICI-related therapy for HCC.
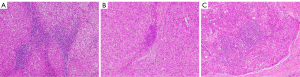
For pathologists, there are no barriers to recognizing the mature TLS, while for the immature TLS without complete germinal center formation, its prognostic value and immune implication are still unapparent accompanied with a poor interobserver consistency (115,116). Therefore, we suggest that the condition of intratumoral mature TLSs is preferential to be reflected in diagnostic report at least. A classification covering the quantity, location, and constitution of intratumoral TLSs in HCC awaits to be drafted. The detailed information of TLSs shall be recorded to accumulate experience for ulterior standardized diagnosis.
PD-L1
It is reasonably expected that the expression of PD-L1 on tumor cells is the underpinning of anti-PD-1 and anti-PD-L1 therapy. The positive stain of PD-L1 by immunohistochemistry is displayed in around 20% of patients of HCC (117). The latest meta-analysis revealed that high PD-L1 expression on tumor tissue represented poorer OS in HCC and was associated with high CD8+ TILs (118,119). The clinicians are undoubtedly pinning their hopes on the great response of ICIs on these patients of HCC with PD-L1 expression. However, the determination of its role was uncertain whatever types of PD-L1 antibodies, platforms for immunohistochemical detection and criteria for judging the positive expression altered across clinical trials (120). Based on the results of KEYNOTE 224, PD-L1 expression (cut-off value ≥1%), as measured by combined positive score (both immune cells and tumor cells with PD-L1 positive expression) instead of tumor proportion score, was relevant to good response to pembrolizumab (121). The results of CHECKMATE 040 and CHECKMATE 459 showed that for patients with PD-L1 expression ≥1% on tumor cells, the proportion of responders in the nivolumab group was higher, but not significantly higher, compared with patients with PD-L1 expression <1% (103,122). The above results make it indefinite in settling PD-L1 into the ICI response prediction for HCC.
For the pathologic assessment, immunohistochemical assays for PD-L1 testing on formalin fixed paraffin embedded tissues have not been authorized as the companion diagnostic to screen patients with HCC likely to benefit from PD-1/PD-L1 inhibitors. We hold on the viewpoint that explorative study should first provide insight into the representativeness of PD-L1 staining on the sections from biopsy or whole tumor, as well as the evaluated cell species, the suitable antibodies, and the positive threshold. Taking non-small cell lung cancer for instance, in order to obtain high concordance with whole sections, four and three biopsied cores were needed at cut-off values of 1% and 50% in the immunohistochemical assessment of PD-L1 (123). PD-L1 expression for non-small cell lung cancer was similar in a single block and three blocks from each resected specimen, indicating that the spatial heterogeneity of PD-L1 expression was within the area represented in one block. Moreover, an excellent intraclass correlation coefficient of accordance for the evaluation of PD-L1 in tumor cells among the pathologists was proved (124). Different antibodies of PD-L1 in determining positive cases showed significant discrepancies at clinically related cut-off values for non-small cell lung cancer (125). To our great knowledge, there is scarcely study to investigate these issues in HCC which makes the formulation of relevant standards with little evidence to follow. Notably, a study from China concluded that PD-L1 antibodies 22C3, 28-8, and SP263 were highly consistent in scoring and affirmed the interchangeability of them. The intraclass correlation coefficient among participating pathologists was moderate to great reliability about the tumor proportion score (126). This finding emerged the basis of the homogenization evaluation of PD-L1 in HCC. Based on the guidelines from Australia (127), Canada (128), and China (129), the immunohistochemical staining of PD-L1 in solid tumors should be separately scored in tumor cells, immune cells, and all cells in order to obtain a series of proportion score. Membranous staining of any intensity should be considered positive for tumor cells. Immune cells are considered positive with any discernible staining. Differ from the algorithm of TIL, multifarious proportion score of PD-L1 is based on the number of tumor/immune cells rather than the area, and the method of area scoring has been merely suggested for urothelial carcinoma to be treated with durvalumab by the SP263 assay (130). The tumor area rather than the whole section should be inspected with different antibodies matching with corresponding threshold values. We suggest that the researches that testing the expression of PD-L1 within the tumor tissue of different regions, morphology, and grading from the same HCC, as well as the tumor with great/poor response of immunotherapy are necessary to be conducted so as to decode the connection between PD-L1 expression and ICI response of HCC.
Wnt/β-catenin
The mutation of CTNNB1 accounts for 11–37% and 0–21% of progressive HCC and early HCC, respectively (131). The correlation between CTNNB1 mutation and prognosis of HCC is still indefinite (132,133). Correlated evidence reminded that activated Wnt/β-catenin signaling characterized the immune evasion across various cancer types and indicated the immunotherapeutic resistance (134). Based on a recent study, the authors exerted a novel genetically engineered mouse model of HCC that enabled interrogating how different genetic alterations influenced on immune surveillance and response to immunotherapies, and they demonstrated that β-catenin activation could block the effect of PD-1 inhibitors (135). This conclusion was further affirmed by the data from an independent cohort and cbioportal webtool that HCC patients with CTNNB1 mutation who receiving ICI therapy were significantly accompanied with suppressed immune cells and increased M2-type macrophages (136). In a small cohort of patients with HCC treated with ICIs, tumors without activated Wnt/β-catenin were more sensitive to ICIs, which leading to better clinical outcomes (137). Conversely, another prospective single center study suggested that the efficacy of atezolizumab plus bevacizumab on patients with HCC might be unaffected by Wnt/β-catenin signal activation (138). Conflicting clinical conclusions hint at a complex interrelationship between CTNNB1 signal pathway and immunotherapy.
For pathologists, the immunohistochemistry of β-catenin is widely used in the diagnosis of various disease, such as fibromatosis, angiofibroma of nasopharynx, solid pseudopapillary tumor of pancreas. In general, the staining of nucleus with >5% tumor cells is interpreted as the activation of Wnt/β-catenin signaling (139). According to our experience, the nuclear expression of β-catenin is usually focal rather than diffuse in HCC, which may result in a misjudgment as false negative (Figure 6A,6B). It is more comprehensive to implement immunohistochemical stain of β-catenin in the wide area and multisite of HCC to avoid erroneous judgement. Furthermore, strong diffuse expression of glutamine synthetase is closely correlated with β-catenin mutation as a meaningful alternative indicator (Figure 6C) (140,141). The technology of DNA sequencing allows us to obtain the information of different point mutations of CTNNB1 gene (142). Remarkably, gene detection of CTNNB1 was not always agreement with protein expression of β-catenin (143), which causes a dilemma whether the lost-budget immunohistochemical staining of β-catenin is able to substitute for the costly genetic testing of CTNNB1 for prediction of immunotherapy in HCC. Moreover, the specific point mutations of CTNNB1 which are strongly related to the immunotherapy of HCC remains to be unraveled.
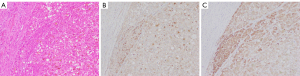
Microsatellite instability and mismatch repair
Microsatellites consist of the repeated sequences of 1–6 nucleotides in the human genome. Mismatch repair (MMR) system comprises several proteins that maintain genome integrity. During the process of DNA replication, the insertion or deletion of repeated units produced by DNA polymerase constantly affect microsatellite stability, while the MMR system is generally able to correct these faults. The malfunction of MMR protein caused by the variation of relate genes, also known as MMR deficiency (dMMR), will result in the aberrant status named as microsatellite instability (MSI) (144). Previously, MSI-high and dMMR were widely used in the colorectal cancer to screen the Lynch syndrome and judge the effectiveness of fluorouracil (145,146). In 2015, the research conducted by Le et al. first confirmed that the dMMR status could predict clinical benefit of treatment with pembrolizumab regardless of tumor types which pioneered a novel predictive biomarker of immunotherapy (147). Therefore, pembrolizumab was granted approval for all MSI-high or dMMR tumors by the US Food and Drug Administration in 2017 and became the first drug of a tumor-agnostic indication (148). This encouraging success promoted a series of explorations on HCC. Unfortunately, the incidence rate of MSI-high/dMMR in HCC was revealed with no more than 5% whether examined by polymerase chain reaction or immunohistochemistry which is highly in inconformity to the actual response rate of ICI-related therapy for HCC (149). Furthermore, a small sample study found that not all patients of HCC within MSI-high status were great responders for pembrolizumab (150). Above negative results imply that MSI-high/dMMR is probably a talentless predictive marker of ICIs for HCC.
Recently, the College of American Pathologists in collaboration with the Association for Molecular Pathology and Fight Colorectal Cancer proposed the guideline of MMR and MSI testing for ICI therapy (151). The guideline recommended that immunohistochemistry for MMR and polymerase chain reaction for MSI rather than next generation sequencing (NGS) assay were still the preferred methods for the examination of DNA MMR defects. The immunohistochemical staining of MLH1, PMS2, MSH2 and MSH6 with complete inexpression of any marker on tumor cells is decided as dMMR. The identification of MSI relies on the markers at 5 loci (Bat-25, Bat-26, D5S346, D17S250, and D2S123), MSI-high if two or more of these markers show instability (i.e., insertion/deletion mutations), MSI-low if only one of the five markers shows instability, and microsatellite stable if all five markers show stability (152). Owing to the extremely low detection rate of dMMR/MSI-high in HCC, we advocate some explorative multicenter researches may be performed firstly in order to accumulate sufficient sample size to determine the response rate of ICI therapy for the HCC with dMMR/MSI-high in the real world, so as to confirm the explicit interrelationship between dMMR/MSI-high and immunotherapy in HCC.
Tumor mutational burden and tumor neoantigen
The presence of abundant somatic mutations in the tumor genome is considered as a valuable feature that responds to ICI therapies, and these mutations are collectively called tumor mutational burden (TMB) (153). These mutations arise mainly due to DNA damage without timely repair resulting in the expression of distorted proteins on tumor cells. These proteins are defined as neoantigen which is nonexistent in normal cells, and can induce an adaptive anti-tumor immune response that is ulteriorly boosted by ICIs (154). Various malignancies including melanoma (155), non-small cell lung cancer (156), and urothelial carcinoma (157) attained an improved response to ICIs when companied with higher TMB and/or neoantigen load. For HCC, TMB burden is generally low with its utility as a biomarker to predict response to ICI unpromising (158). Cai et al. put forward that higher TMB was associated with adverse prognosis with a cut-off value of 4.8 mutations/Mb based on the 128 patients of HCC who underwent radical hepatectomy (159). However, Liu et al. considered that a higher TMB level indicated better OS for HCC and was correlated with early pathological stages based on The Cancer Genome Atlas database (160). Analogously, Liu et al. certified that patients with HCC had better OS when possessed high-affinity neoantigens value (161). Mauriello et al. concluded that high neoantigen burden was uncorrelated with decreased progression-free survival in HCC patients (162). These paradoxical prognostic results also impede their applications in immunotherapy.
For pathologists, the above conclusions raise two important questions: (I) how to identify the optimal cut-off value of TMB for stratifying immunotherapeutic response of HCC; (II) how to distinguish the immunogenic neoantigens from genomic data. For the first question, based on the KEYNOTE-158 trial, the US Food and Drug Administration ratified that the cancers with TMB of at least 10 mutations/Mb was postulated to adopt pembrolizumab so as to reduce healthcare disparities by broadly expanding treatment eligibility (163,164). However, this criterion is still incongruous for HCC as its low TMB. A more appropriate cut-off value of TMB for HCC needs to be further explored and determined. For the second question, computational neoantigen prediction traditionally focuses on peptides binding to major histocompatibility complex on account of anchor residue identities. Nevertheless, neoantigen burden determined by this method usually poorly forecasts the ICI response and survival, in addition it is quite poor for the positive predictive value of these predictions in functional assays (165). NGS is the only technique to harbor TMB and neoantigen. Its expensive cost and inconsistent platforms restrict extensive applications in the routine pathologic work.
Some other signaling pathways related to immunotherapy
With the development of omics, some signaling pathways related to the immunotherapy of HCC have been discovered, and their alternations may indicate the therapeutic resistance of ICIs (165,166). For instance, the loss-of-function mutations of Janus tyrosine kinases 1 and 2 (JAK-1 and JAK-2) is a noteworthy cause of the resistance to ICIs as the integrity of these kinases is essential for interferon-α intracellular signaling which is critical in the priming of T cells by antigen presenting cells (167,168). Phosphatase and tensin homologue (PTEN) knock-down decreases the ability of T cells to kill tumor cells through the indirect activation of signal transducer and activator of transcription 3 (STAT3) which is a crucial positive regulator of immune evasion (169). As such, PTEN-STAT3 pathway is also a meaningful pathway in immunotherapy and nearly 60% of human HCCs exhibit activated nuclear STAT3 (170). Dysregulated signaling of transforming growth factor beta (TGF-β) pathway plays a central part in immunomodulation of the TME of HCC (171). On the one hand, tumor cells and all stromal cells modulate Treg cell activity via this pathway (171). On the other hand, increased TGF-β signaling may give rise to T cell exhaustion by upregulation of PD-1 signaling (172). Inhibition of TGF-β signaling may directly enhance antitumor immunity in HCC.
It is frustrating that the variations of all above signaling pathways could only be determined by NGS of which the high cost and complex analysis strategies obstruct their applications. We believe that the development of highly sensitive and specific antibodies of these molecular pathways may be optimal to solve these issues.
Conclusions
The clinical management of HCC in the era of immunotherapy is giving birth to two brand-new pathological diagnosis concerns, those are, how to reasonably evaluate the efficacy of immunotherapy and how to accurately predict the potential of immunotherapy, by which will furnish the most reliable evidence for screening the patients of HCC suitable for immunotherapy. The growing clinical needs urge pathologists to face up to and solve these issues. Defectively, the standard pathologic evaluation of HCC underwent immunotherapy is limited by the huge tumor body, extremely assorted pathologic changes, and complex immune microenvironment. An ample and moderate sampling range should be exactly designed so as to accurately reconstruct the imaging of immunotherapy. Not only the area of viable tumor, necrosis, and fibrosis should be precisely quantified, but also the typing of residual tumor nests, morphology and phenotype of visible tumor, pattern of necrosis, characteristics of stromal reaction, as well as the pathological condition of surrounding liver tissue should be of great concerned. In regard to the prediction of immunotherapeutic efficacy of HCC, the proposed markers from numerous studies are fraught with controversy due to the multidimensional intratumoral heterogeneity and multiple pathways in tumorigenesis. Through the histomorphology, immunohistochemistry, and polymerase chain reaction, the testing and evaluation of TIL, TLS, PD-L1 expression, Wnt/β-catenin mutation, as well as MSI and MMR is workable. The popularity of NGS technology makes it possible to examine TMB and neoantigen, as well as another related molecular pathway. Although the deep sequencing technology could help us panoramically explore the mechanism of immunotherapy of HCC, whereas the high cost restricts it popularize in routine work, meanwhile its huge dataset challenges the further dimensional reduction analysis. We believe that pathologists still require to focus on the conventional pathology and gradually form a highly reproducible evaluation strategy for the effect forecast of immunotherapy for HCC, and the deep sequencing is a powerful verification means. Where there is light, there is hope. We need to be down-to-earth and bring more gospel for such patients.
Acknowledgments
Funding: We have received funding from
Footnote
Reporting Checklist: The authors have completed the Narrative Review reporting checklist. Available at https://hbsn.amegroups.com/article/view/10.21037/hbsn-22-527/rc
Conflicts of Interest: All authors have completed the ICMJE uniform disclosure form (available at https://hbsn.amegroups.com/article/view/10.21037/hbsn-22-527/coif). The authors have no conflicts of interest to declare.
Ethical Statement: The authors are accountable for all aspects of the work in ensuring that questions related to the accuracy or integrity of any part of the work are appropriately investigated and resolved.
Open Access Statement: This is an Open Access article distributed in accordance with the Creative Commons Attribution-NonCommercial-NoDerivs 4.0 International License (CC BY-NC-ND 4.0), which permits the non-commercial replication and distribution of the article with the strict proviso that no changes or edits are made and the original work is properly cited (including links to both the formal publication through the relevant DOI and the license). See: https://creativecommons.org/licenses/by-nc-nd/4.0/.
References
- Xia C, Dong X, Li H, et al. Cancer statistics in China and United States, 2022: profiles, trends, and determinants. Chin Med J (Engl) 2022;135:584-90. [Crossref] [PubMed]
- Sung H, Ferlay J, Siegel RL, et al. Global Cancer Statistics 2020: GLOBOCAN Estimates of Incidence and Mortality Worldwide for 36 Cancers in 185 Countries. CA Cancer J Clin 2021;71:209-49. [Crossref] [PubMed]
- Fu Y, Liu S, Zeng S, et al. From bench to bed: the tumor immune microenvironment and current immunotherapeutic strategies for hepatocellular carcinoma. J Exp Clin Cancer Res 2019;38:396. [Crossref] [PubMed]
- Huang A, Yang XR, Chung WY, et al. Targeted therapy for hepatocellular carcinoma. Signal Transduct Target Ther 2020;5:146. [Crossref] [PubMed]
- Yang Y. Cancer immunotherapy: harnessing the immune system to battle cancer. J Clin Invest 2015;125:3335-7. [Crossref] [PubMed]
- Lee HT, Lee SH, Heo YS. Molecular Interactions of Antibody Drugs Targeting PD-1, PD-L1, and CTLA-4 in Immuno-Oncology. Molecules 2019;24:1190. [Crossref] [PubMed]
- Freeman GJ, Long AJ, Iwai Y, et al. Engagement of the PD-1 immunoinhibitory receptor by a novel B7 family member leads to negative regulation of lymphocyte activation. J Exp Med 2000;192:1027-34. [Crossref] [PubMed]
- Sun C, Mezzadra R, Schumacher TN. Regulation and Function of the PD-L1 Checkpoint. Immunity 2018;48:434-52. [Crossref] [PubMed]
- Mitsuiki N, Schwab C, Grimbacher B. What did we learn from CTLA-4 insufficiency on the human immune system? Immunol Rev 2019;287:33-49. [Crossref] [PubMed]
- Qureshi OS, Zheng Y, Nakamura K, et al. Trans-endocytosis of CD80 and CD86: a molecular basis for the cell-extrinsic function of CTLA-4. Science 2011;332:600-3. [Crossref] [PubMed]
- Kumar P, Saini S, Prabhakar BS. Cancer immunotherapy with check point inhibitor can cause autoimmune adverse events due to loss of Treg homeostasis. Semin Cancer Biol 2020;64:29-35. [Crossref] [PubMed]
- Ghavimi S, Apfel T, Azimi H, et al. Management and Treatment of Hepatocellular Carcinoma with Immunotherapy: A Review of Current and Future Options. J Clin Transl Hepatol 2020;8:168-76. [Crossref] [PubMed]
- Tumeh PC, Harview CL, Yearley JH, et al. PD-1 blockade induces responses by inhibiting adaptive immune resistance. Nature 2014;515:568-71. [Crossref] [PubMed]
- Morad G, Helmink BA, Sharma P, et al. Hallmarks of response, resistance, and toxicity to immune checkpoint blockade. Cell 2021;184:5309-37. [Crossref] [PubMed]
- Chinese Chapter of the International Hepato-Pancreato-Biliary Association. Expert Committee on Liver Cancer, Chinese Society of Clinical Oncology. Chinese multidisciplinary expert consensus on combined immunotherapy based on immune checkpoint inhibitors for hepatocellular carcinoma (2021 version). Zhonghua Gan Zang Bing Za Zhi 2021;29:636-47. [PubMed]
- Hepatobiliary cancers, Version 2.2022, NCCN clinical practice guidelines in oncology. July 15, 2022. Available online: https://www.nccn.org/guidelines/guidelines-detail?category=1&id=1438
- Zhao HT, Cai JQ. Chinese expert consensus on neoadjuvant and conversion therapies for hepatocellular carcinoma. World J Gastroenterol 2021;27:8069-80. [Crossref] [PubMed]
- Sun HC, Zhou J, Wang Z, et al. Chinese expert consensus on conversion therapy for hepatocellular carcinoma (2021 edition). Hepatobiliary Surg Nutr 2022;11:227-52. [Crossref] [PubMed]
- Seymour L, Bogaerts J, Perrone A, et al. iRECIST: guidelines for response criteria for use in trials testing immunotherapeutics. Lancet Oncol 2017;18:e143-52. [Crossref] [PubMed]
- Lencioni R, Llovet JM. Modified RECIST (mRECIST) assessment for hepatocellular carcinoma. Semin Liver Dis 2010;30:52-60. [Crossref] [PubMed]
- Llovet JM, Lencioni R. mRECIST for HCC: Performance and novel refinements. J Hepatol 2020;72:288-306. [Crossref] [PubMed]
- Lee CK, Chan SL, Chon HJ. Could We Predict the Response of Immune Checkpoint Inhibitor Treatment in Hepatocellular Carcinoma? Cancers (Basel) 2022;14:3213. [Crossref] [PubMed]
- Zhou WP, Lai EC, Li AJ, et al. A prospective, randomized, controlled trial of preoperative transarterial chemoembolization for resectable large hepatocellular carcinoma. Ann Surg 2009;249:195-202. [Crossref] [PubMed]
- Wei X, Jiang Y, Zhang X, et al. Neoadjuvant Three-Dimensional Conformal Radiotherapy for Resectable Hepatocellular Carcinoma With Portal Vein Tumor Thrombus: A Randomized, Open-Label, Multicenter Controlled Study. J Clin Oncol 2019;37:2141-51. [Crossref] [PubMed]
- Pinato DJ, Fessas P, Sapisochin G, et al. Perspectives on the Neoadjuvant Use of Immunotherapy in Hepatocellular Carcinoma. Hepatology 2021;74:483-90. [Crossref] [PubMed]
- Marron TU, Schwartz M, Corbett V, et al. Neoadjuvant Immunotherapy for Hepatocellular Carcinoma. J Hepatocell Carcinoma 2022;9:571-81. [Crossref] [PubMed]
- Ho WJ, Zhu Q, Durham J, et al. Neoadjuvant Cabozantinib and Nivolumab Converts Locally Advanced HCC into Resectable Disease with Enhanced Antitumor Immunity. Nat Cancer 2021;2:891-903. [Crossref] [PubMed]
- Marron TU, Fiel MI, Hamon P, et al. Neoadjuvant cemiplimab for resectable hepatocellular carcinoma: a single-arm, open-label, phase 2 trial. Lancet Gastroenterol Hepatol 2022;7:219-29. [Crossref] [PubMed]
- Kaseb AO, Hasanov E, Cao HST, et al. Perioperative nivolumab monotherapy versus nivolumab plus ipilimumab in resectable hepatocellular carcinoma: a randomised, open-label, phase 2 trial. Lancet Gastroenterol Hepatol 2022;7:208-18. [Crossref] [PubMed]
- Xia Y, Tang W, Qian X, et al. Efficacy and safety of camrelizumab plus apatinib during the perioperative period in resectable hepatocellular carcinoma: a single-arm, open label, phase II clinical trial. J Immunother Cancer 2022;10:e004656. [Crossref] [PubMed]
- Zhu XD, Huang C, Shen YH, et al. Downstaging and Resection of Initially Unresectable Hepatocellular Carcinoma with Tyrosine Kinase Inhibitor and Anti-PD-1 Antibody Combinations. Liver Cancer 2021;10:320-9. [Crossref] [PubMed]
- Bruix J, Chan SL, Galle PR, et al. Systemic treatment of hepatocellular carcinoma: An EASL position paper. J Hepatol 2021;75:960-74. [Crossref] [PubMed]
- Heimbach JK, Kulik LM, Finn RS, et al. AASLD guidelines for the treatment of hepatocellular carcinoma. Hepatology 2018;67:358-80. [Crossref] [PubMed]
- Burt AD, Alves V, Clouston AD, Guido M, Hübscher SG, Kakar S, et al. Intrahepatic cholangiocarcinoma, perihilar cholangiocarcinoma and hepatocellular carcinoma histopathology reporting guide. International Collaboration on Cancer Reporting. November, 2020. Available online: https://www.iccr-cancer.org/datasets/published-datasets/digestive-tract/liver/
- Protocol for the examination of specimens from patients with hepatocellular carcinoma. College of American Pathologists. February 1, 2011. Available online: https://documents.cap.org/protocols/cp-hepatocellular-carcinoma-2012-v3100.pdf
- Sheng X, Ji Y, Ren GP, et al. A standardized pathological proposal for evaluating microvascular invasion of hepatocellular carcinoma: a multicenter study by LCPGC. Hepatol Int 2020;14:1034-47. [Crossref] [PubMed]
- Zhou J, Sun H, Wang Z, et al. Guidelines for the Diagnosis and Treatment of Hepatocellular Carcinoma (2019 Edition). Liver Cancer 2020;9:682-720. [Crossref] [PubMed]
- Weissferdt A, Pataer A, Swisher SG, et al. Controversies and challenges in the pathologic examination of lung resection specimens after neoadjuvant treatment. Lung Cancer 2021;154:76-83. [Crossref] [PubMed]
- Xue Y, Farris AB, Quigley B, et al. The Impact of New Technologic and Molecular Advances in the Daily Practice of Gastrointestinal and Hepatobiliary Pathology. Arch Pathol Lab Med 2017;141:517-27. [Crossref] [PubMed]
- Provenzano E, Bossuyt V, Viale G, et al. Standardization of pathologic evaluation and reporting of postneoadjuvant specimens in clinical trials of breast cancer: recommendations from an international working group. Mod Pathol 2015;28:1185-201. [Crossref] [PubMed]
- Bossuyt V, Symmans WF. Standardizing of Pathology in Patients Receiving Neoadjuvant Chemotherapy. Ann Surg Oncol 2016;23:3153-61. [Crossref] [PubMed]
- Cottrell TR, Thompson ED, Forde PM, et al. Pathologic features of response to neoadjuvant anti-PD-1 in resected non-small-cell lung carcinoma: a proposal for quantitative immune-related pathologic response criteria (irPRC). Ann Oncol 2018;29:1853-60. [Crossref] [PubMed]
- Travis WD, Dacic S, Wistuba I, et al. IASLC Multidisciplinary Recommendations for Pathologic Assessment of Lung Cancer Resection Specimens After Neoadjuvant Therapy. J Thorac Oncol 2020;15:709-40. [Crossref] [PubMed]
- Wang H, Chetty R, Hosseini M, et al. Pathologic Examination of Pancreatic Specimens Resected for Treated Pancreatic Ductal Adenocarcinoma: Recommendations From the Pancreatobiliary Pathology Society. Am J Surg Pathol 2022;46:754-64. [Crossref] [PubMed]
- Stein JE, Lipson EJ, Cottrell TR, et al. Pan-Tumor Pathologic Scoring of Response to PD-(L)1 Blockade. Clin Cancer Res 2020;26:545-51. [Crossref] [PubMed]
- Pathological Complete Response in neoadjuvant treatment of high-risk early-stage breast cancer: use as an endpoint to support accelerated approval guidance for industry. the U.S. Food and Drug Administration. July 2020. Available online: https://www.fda.gov/media/83507/download
- Quirke P, Durdey P, Dixon MF, et al. Local recurrence of rectal adenocarcinoma due to inadequate surgical resection. Histopathological study of lateral tumour spread and surgical excision. Lancet 1986;2:996-9. [Crossref] [PubMed]
- Tetzlaff MT, Messina JL, Stein JE, et al. Pathological assessment of resection specimens after neoadjuvant therapy for metastatic melanoma. Ann Oncol 2018;29:1861-8. [Crossref] [PubMed]
- Yang Y, Dang Z, Lu P, et al. Impact of pathological response after preoperative transcatheter arterial chemoembolization (TACE) on incidences of microvascular invasion and early tumor recurrence in hepatocellular carcinoma: a multicenter propensity score matching analysis. Hepatobiliary Surg Nutr 2022;11:386-99. [Crossref] [PubMed]
- Zhou N, Velez MA, Owen D, et al. Immune-Related Adverse Events (irAEs): Implications for Immune Checkpoint Inhibitor Therapy. J Natl Compr Canc Netw 2020;18:1287-90. [Crossref] [PubMed]
- Bagchi S, Yuan R, Engleman EG. Immune Checkpoint Inhibitors for the Treatment of Cancer: Clinical Impact and Mechanisms of Response and Resistance. Annu Rev Pathol 2021;16:223-49. [Crossref] [PubMed]
- Morisco F, Stigliano R, Godfrey A, et al. Efficacy of loco-regional ablation therapy of HCC in a population of liver transplanted patients. Dig Dis Sci 2008;53:1131-7. [Crossref] [PubMed]
- Cotoi CG, Khorsandi SE, Pleşea IE, et al. Histological aspects of post-TACE hepatocellular carcinoma. Rom J Morphol Embryol 2012;53:677-82. [PubMed]
- Sakon M, Monden M, Umeshita K, et al. The prognostic significance of macroscopic growth pattern of hepatocellular carcinoma. Int Surg 1994;79:38-42. [PubMed]
- Rich NE, John BV, Parikh ND, et al. Hepatocellular Carcinoma Demonstrates Heterogeneous Growth Patterns in a Multicenter Cohort of Patients With Cirrhosis. Hepatology 2020;72:1654-65. [Crossref] [PubMed]
- Matoso A, Parimi V, Epstein JI. Noninvasive low-grade papillary urothelial carcinoma with degenerative nuclear atypia: a grading pitfall. Hum Pathol 2021;113:1-8. [Crossref] [PubMed]
- Nishihara Y, Aishima S, Kuroda Y, et al. Biliary phenotype of hepatocellular carcinoma after preoperative transcatheter arterial chemoembolization. J Gastroenterol Hepatol 2008;23:1860-8. [Crossref] [PubMed]
- Le T, Shahriari P, Hopkins L, et al. Prognostic significance of tumor necrosis in ovarian cancer patients treated with neoadjuvant chemotherapy and interval surgical debulking. Int J Gynecol Cancer 2006;16:986-90. [Crossref] [PubMed]
- Pataer A, Kalhor N, Correa AM, et al. Histopathologic response criteria predict survival of patients with resected lung cancer after neoadjuvant chemotherapy. J Thorac Oncol 2012;7:825-32. [Crossref] [PubMed]
- Kudo M, Ishii G, Gotohda N, et al. Histological tumor necrosis in pancreatic cancer after neoadjuvant therapy. Oncol Rep 2022;48:121. [Crossref] [PubMed]
- Wu ZJ, Xie YF, Chang X, et al. Type of Necrosis Influences Prognosis in Hepatocellular Carcinoma After the First Transarterial Chemoembolization. Med Sci Monit 2021;27:e929884. [Crossref] [PubMed]
- Wang Y, Zhou C, Liu J, et al. Increased Liquefactive Necrosis Formation After Transarterial Chemoembolization Combined with Molecular Targeted Agents Plus Immune Checkpoint Inhibitors for Hepatocellular Carcinoma. Cancer Manag Res 2021;13:6935-41. [Crossref] [PubMed]
- Lu C, Rong D, Zhang B, et al. Current perspectives on the immunosuppressive tumor microenvironment in hepatocellular carcinoma: challenges and opportunities. Mol Cancer 2019;18:130. [Crossref] [PubMed]
- Santhakumar C, Gane EJ, Liu K, et al. Current perspectives on the tumor microenvironment in hepatocellular carcinoma. Hepatol Int 2020;14:947-57. [Crossref] [PubMed]
- Xiang S, Li J, Shen J, et al. Identification of Prognostic Genes in the Tumor Microenvironment of Hepatocellular Carcinoma. Front Immunol 2021;12:653836. [Crossref] [PubMed]
- Tian Y, Abu-Sbeih H, Wang Y. Immune Checkpoint Inhibitors-Induced Hepatitis. Adv Exp Med Biol 2018;995:159-64. [Crossref] [PubMed]
- Topalian SL, Sznol M, McDermott DF, et al. Survival, durable tumor remission, and long-term safety in patients with advanced melanoma receiving nivolumab. J Clin Oncol 2014;32:1020-30. [Crossref] [PubMed]
- Bernardo SG, Moskalenko M, Pan M, et al. Elevated rates of transaminitis during ipilimumab therapy for metastatic melanoma. Melanoma Res 2013;23:47-54. [Crossref] [PubMed]
- Larkin J, Chiarion-Sileni V, Gonzalez R, et al. Combined Nivolumab and Ipilimumab or Monotherapy in Untreated Melanoma. N Engl J Med 2015;373:23-34. [Crossref] [PubMed]
- De Martin E, Michot JM, Papouin B, et al. Characterization of liver injury induced by cancer immunotherapy using immune checkpoint inhibitors. J Hepatol 2018;68:1181-90. [Crossref] [PubMed]
- Zen Y, Yeh MM. Hepatotoxicity of immune checkpoint inhibitors: a histology study of seven cases in comparison with autoimmune hepatitis and idiosyncratic drug-induced liver injury. Mod Pathol 2018;31:965-73. [Crossref] [PubMed]
- Johncilla M, Misdraji J, Pratt DS, et al. Ipilimumab-associated Hepatitis: Clinicopathologic Characterization in a Series of 11 Cases. Am J Surg Pathol 2015;39:1075-84. [Crossref] [PubMed]
- Simoes CC, Thung SN, Fiel MI, et al. Morphology of tumor and nontumor tissue in liver resection specimens for hepatocellular carcinoma following nivolumab therapy. Mod Pathol 2021;34:823-33. [Crossref] [PubMed]
- Peeraphatdit TB, Wang J, Odenwald MA, et al. Hepatotoxicity From Immune Checkpoint Inhibitors: A Systematic Review and Management Recommendation. Hepatology 2020;72:315-29. [Crossref] [PubMed]
- Berruti A, Amoroso V, Gallo F, et al. Pathologic complete response as a potential surrogate for the clinical outcome in patients with breast cancer after neoadjuvant therapy: a meta-regression of 29 randomized prospective studies. J Clin Oncol 2014;32:3883-91. [Crossref] [PubMed]
- Menzies AM, Amaria RN, Rozeman EA, et al. Pathological response and survival with neoadjuvant therapy in melanoma: a pooled analysis from the International Neoadjuvant Melanoma Consortium (INMC). Nat Med 2021;27:301-9. [Crossref] [PubMed]
- Hellmann MD, Chaft JE, William WN Jr, et al. Pathological response after neoadjuvant chemotherapy in resectable non-small-cell lung cancers: proposal for the use of major pathological response as a surrogate endpoint. Lancet Oncol 2014;15:e42-50. [Crossref] [PubMed]
- Langer R, Becker K. Tumor regression grading of gastrointestinal cancers after neoadjuvant therapy. Virchows Arch 2018;472:175-86. [Crossref] [PubMed]
- Schreiner W, Dudek W, Rieker RJ, et al. Major Pathologic Response after Induction Therapy Has a Long-Term Impact on Survival and Tumor Recurrence in Stage IIIA/B Locally Advanced NSCLC. Thorac Cardiovasc Surg 2020;68:639-45. [Crossref] [PubMed]
- Chalabi M, Fanchi LF, Dijkstra KK, et al. Neoadjuvant immunotherapy leads to pathological responses in MMR-proficient and MMR-deficient early-stage colon cancers. Nat Med 2020;26:566-76. [Crossref] [PubMed]
- Stein JE, Soni A, Danilova L, et al. Major pathologic response on biopsy (MPRbx) in patients with advanced melanoma treated with anti-PD-1: evidence for an early, on-therapy biomarker of response. Ann Oncol 2019;30:589-96. [Crossref] [PubMed]
- Bureau of Medical Administration, National Health Commission of the People's Republic of China. Standardization for diagnosis and treatment of hepatocellular carcinoma (2022 edition). Zhonghua Gan Zang Bing Za Zhi 2022;30:367-88. [PubMed]
- Ding G, Guo M, Yang Y, et al. Large-Section Histopathology Can Better Indicate the Immune Microenvironment and Predict the Prognosis of Pancreatic Ductal Adenocarcinoma Than Small-Section Histopathology. Front Oncol 2021;11:694933. [Crossref] [PubMed]
- Saillard C, Schmauch B, Laifa O, et al. Predicting Survival After Hepatocellular Carcinoma Resection Using Deep Learning on Histological Slides. Hepatology 2020;72:2000-13. [Crossref] [PubMed]
- Zeng Q, Klein C, Caruso S, et al. Artificial intelligence predicts immune and inflammatory gene signatures directly from hepatocellular carcinoma histology. J Hepatol 2022;77:116-27. [Crossref] [PubMed]
- Pinter M, Jain RK, Duda DG. The Current Landscape of Immune Checkpoint Blockade in Hepatocellular Carcinoma: A Review. JAMA Oncol 2021;7:113-23. [Crossref] [PubMed]
- Gibney GT, Weiner LM, Atkins MB. Predictive biomarkers for checkpoint inhibitor-based immunotherapy. Lancet Oncol 2016;17:e542-51. [Crossref] [PubMed]
- Rizzo A, Ricci AD, Di Federico A, et al. Predictive Biomarkers for Checkpoint Inhibitor-Based Immunotherapy in Hepatocellular Carcinoma: Where Do We Stand? Front Oncol 2021;11:803133. [Crossref] [PubMed]
- Heinrich S, Craig AJ, Ma L, et al. Understanding tumour cell heterogeneity and its implication for immunotherapy in liver cancer using single-cell analysis. J Hepatol 2021;74:700-15. [Crossref] [PubMed]
- Friemel J, Rechsteiner M, Frick L, et al. Intratumor heterogeneity in hepatocellular carcinoma. Clin Cancer Res 2015;21:1951-61. [Crossref] [PubMed]
- Losic B, Craig AJ, Villacorta-Martin C, et al. Intratumoral heterogeneity and clonal evolution in liver cancer. Nat Commun 2020;11:291. [Crossref] [PubMed]
- Bruni D, Angell HK, Galon J. The immune contexture and Immunoscore in cancer prognosis and therapeutic efficacy. Nat Rev Cancer 2020;20:662-80. [Crossref] [PubMed]
- Fu J, Xu D, Liu Z, et al. Increased regulatory T cells correlate with CD8 T-cell impairment and poor survival in hepatocellular carcinoma patients. Gastroenterology 2007;132:2328-39. [Crossref] [PubMed]
- Zhang JP, Yan J, Xu J, et al. Increased intratumoral IL-17-producing cells correlate with poor survival in hepatocellular carcinoma patients. J Hepatol 2009;50:980-9. [Crossref] [PubMed]
- Gao F, Xie K, Xiang Q, et al. The density of tumor-infiltrating lymphocytes and prognosis in resectable hepatocellular carcinoma: a two-phase study. Aging (Albany NY) 2021;13:9665-78. [Crossref] [PubMed]
- Zheng X, Jin W, Wang S, et al. Progression on the Roles and Mechanisms of Tumor-Infiltrating T Lymphocytes in Patients With Hepatocellular Carcinoma. Front Immunol 2021;12:729705. [Crossref] [PubMed]
- Zhang L, Zhang W, Li Z, et al. Mitochondria dysfunction in CD8+ T cells as an important contributing factor for cancer development and a potential target for cancer treatment: a review. J Exp Clin Cancer Res 2022;41:227. [Crossref] [PubMed]
- Whiteside TL, Schuler P, Schilling B. Induced and natural regulatory T cells in human cancer. Expert Opin Biol Ther 2012;12:1383-97. [Crossref] [PubMed]
- Schoenberg MB, Hao J, Bucher JN, et al. Perivascular Tumor-Infiltrating Leukocyte Scoring for Prognosis of Resected Hepatocellular Carcinoma Patients. Cancers (Basel) 2018;10:389. [Crossref] [PubMed]
- Huang Y, Wang FM, Wang T, et al. Tumor-infiltrating FoxP3+ Tregs and CD8+ T cells affect the prognosis of hepatocellular carcinoma patients. Digestion 2012;86:329-37. [Crossref] [PubMed]
- Wang F, Jing X, Li G, et al. Foxp3+ regulatory T cells are associated with the natural history of chronic hepatitis B and poor prognosis of hepatocellular carcinoma. Liver Int 2012;32:644-55. [Crossref] [PubMed]
- Zhang Y, Zhang Z. The history and advances in cancer immunotherapy: understanding the characteristics of tumor-infiltrating immune cells and their therapeutic implications. Cell Mol Immunol 2020;17:807-21. [Crossref] [PubMed]
- Sangro B, Melero I, Wadhawan S, et al. Association of inflammatory biomarkers with clinical outcomes in nivolumab-treated patients with advanced hepatocellular carcinoma. J Hepatol 2020;73:1460-9. [Crossref] [PubMed]
- Duffy AG, Ulahannan SV, Makorova-Rusher O, et al. Tremelimumab in combination with ablation in patients with advanced hepatocellular carcinoma. J Hepatol 2017;66:545-51. [Crossref] [PubMed]
- Zhu AX, Abbas AR, de Galarreta MR, et al. Molecular correlates of clinical response and resistance to atezolizumab in combination with bevacizumab in advanced hepatocellular carcinoma. Nat Med 2022;28:1599-611. [Crossref] [PubMed]
- D'Alessio A, Pinato DJ. Dissecting the Tumor Microenvironment to Predict Immunotherapy Response in Hepatocellular Cancer. Gastroenterology 2022;163:1712-3. [Crossref] [PubMed]
- Kumagai S, Togashi Y, Kamada T, et al. The PD-1 expression balance between effector and regulatory T cells predicts the clinical efficacy of PD-1 blockade therapies. Nat Immunol 2020;21:1346-58. [Crossref] [PubMed]
- Kim HD, Song GW, Park S, et al. Association Between Expression Level of PD1 by Tumor-Infiltrating CD8(+) T Cells and Features of Hepatocellular Carcinoma. Gastroenterology 2018;155:1936-1950.e17. [Crossref] [PubMed]
- Haber PK, Castet F, Torres-Martin M, et al. Molecular Markers of Response to Anti-PD1 Therapy in Advanced Hepatocellular Carcinoma. Gastroenterology 2023;164:72-88.e18. [Crossref] [PubMed]
- Brandi G, Tovoli F, Tavolari S. Have We Found the "Holy Grail" That May Predict Response to Immunotherapy in Hepatocellular Carcinoma? Gastroenterology 2023;164:15-8. [Crossref] [PubMed]
- Salgado R, Denkert C, Demaria S, et al. The evaluation of tumor-infiltrating lymphocytes (TILs) in breast cancer: recommendations by an International TILs Working Group 2014. Ann Oncol 2015;26:259-71. [Crossref] [PubMed]
- Hendry S, Salgado R, Gevaert T, et al. Assessing Tumor-Infiltrating Lymphocytes in Solid Tumors: A Practical Review for Pathologists and Proposal for a Standardized Method from the International Immuno-Oncology Biomarkers Working Group: Part 2: TILs in Melanoma, Gastrointestinal Tract Carcinomas, Non-Small Cell Lung Carcinoma and Mesothelioma, Endometrial and Ovarian Carcinomas, Squamous Cell Carcinoma of the Head and Neck, Genitourinary Carcinomas, and Primary Brain Tumors. Adv Anat Pathol 2017;24:311-35. [Crossref] [PubMed]
- Teillaud JL, Dieu-Nosjean MC. Tertiary Lymphoid Structures: An Anti-tumor School for Adaptive Immune Cells and an Antibody Factory to Fight Cancer? Front Immunol 2017;8:830. [Crossref] [PubMed]
- Sautès-Fridman C, Petitprez F, Calderaro J, et al. Tertiary lymphoid structures in the era of cancer immunotherapy. Nat Rev Cancer 2019;19:307-25. [Crossref] [PubMed]
- Calderaro J, Petitprez F, Becht E, et al. Intra-tumoral tertiary lymphoid structures are associated with a low risk of early recurrence of hepatocellular carcinoma. J Hepatol 2019;70:58-65. [Crossref] [PubMed]
- Meylan M, Petitprez F, Lacroix L, et al. Early Hepatic Lesions Display Immature Tertiary Lymphoid Structures and Show Elevated Expression of Immune Inhibitory and Immunosuppressive Molecules. Clin Cancer Res 2020;26:4381-9. [Crossref] [PubMed]
- Calderaro J, Rousseau B, Amaddeo G, et al. Programmed death ligand 1 expression in hepatocellular carcinoma: Relationship With clinical and pathological features. Hepatology 2016;64:2038-46. [Crossref] [PubMed]
- Zhou ZY, Liu SR, Xu LB, et al. Clinicopathological and Prognostic Value of Programmed Cell Death 1 Expression in Hepatitis B Virus-related Hepatocellular Carcinoma: A Meta-analysis. J Clin Transl Hepatol 2021;9:889-97. [Crossref] [PubMed]
- Li XS, Li JW, Li H, et al. Prognostic value of programmed cell death ligand 1 (PD-L1) for hepatocellular carcinoma: a meta-analysis. Biosci Rep 2020;40:BSR20200459. [Crossref] [PubMed]
- Wang X, Teng F, Kong L, et al. PD-L1 expression in human cancers and its association with clinical outcomes. Onco Targets Ther 2016;9:5023-39. [Crossref] [PubMed]
- Zhu AX, Finn RS, Edeline J, et al. Pembrolizumab in patients with advanced hepatocellular carcinoma previously treated with sorafenib (KEYNOTE-224): a non-randomised, open-label phase 2 trial. Lancet Oncol 2018;19:940-52. [Crossref] [PubMed]
- Yau T, Park JW, Finn RS, et al. Nivolumab versus sorafenib in advanced hepatocellular carcinoma (CheckMate 459): a randomised, multicentre, open-label, phase 3 trial. Lancet Oncol 2022;23:77-90. [Crossref] [PubMed]
- Munari E, Zamboni G, Lunardi G, et al. PD-L1 Expression Heterogeneity in Non-Small Cell Lung Cancer: Defining Criteria for Harmonization between Biopsy Specimens and Whole Sections. J Thorac Oncol 2018;13:1113-20. [Crossref] [PubMed]
- Rehman JA, Han G, Carvajal-Hausdorf DE, et al. Quantitative and pathologist-read comparison of the heterogeneity of programmed death-ligand 1 (PD-L1) expression in non-small cell lung cancer. Mod Pathol 2017;30:340-9. [Crossref] [PubMed]
- Rimm DL, Han G, Taube JM, et al. A Prospective, Multi-institutional, Pathologist-Based Assessment of 4 Immunohistochemistry Assays for PD-L1 Expression in Non-Small Cell Lung Cancer. JAMA Oncol 2017;3:1051-8. [Crossref] [PubMed]
- Shi L, Zhang SJ, Chen J, et al. A comparability study of immunohistochemical assays for PD-L1 expression in hepatocellular carcinoma. Mod Pathol 2019;32:1646-56. [Crossref] [PubMed]
- Paver EC, Cooper WA, Colebatch AJ, et al. Programmed death ligand-1 (PD-L1) as a predictive marker for immunotherapy in solid tumours: a guide to immunohistochemistry implementation and interpretation. Pathology 2021;53:141-56. [Crossref] [PubMed]
- Cheung CC, Barnes P, Bigras G, et al. Fit-For-Purpose PD-L1 Biomarker Testing For Patient Selection in Immuno-Oncology: Guidelines For Clinical Laboratories From the Canadian Association of Pathologists-Association Canadienne Des Pathologistes (CAP-ACP). Appl Immunohistochem Mol Morphol 2019;27:699-714. [Crossref] [PubMed]
- Pathology Quality Control Center. Pathology Committee of Chinese Society of Clinical Oncology. Consensus on the immunohistochemical tests of PD-L1 in solid tumors (2021 version). Zhonghua Bing Li Xue Za Zhi 2021;50:710-8.
- Eckstein M, Cimadamore A, Hartmann A, et al. PD-L1 assessment in urothelial carcinoma: a practical approach. Ann Transl Med 2019;7:690. [Crossref] [PubMed]
- Torbenson M, Ng I, Park Y, et al. Hepatocellular carcinoma. WHO classification of tumours. Digestive system tumours. 5th edition: Lyon: IARC Press; 2019:229-239.
- Chen J, Liu J, Jin R, et al. Cytoplasmic and/or nuclear expression of β-catenin correlate with poor prognosis and unfavorable clinicopathological factors in hepatocellular carcinoma: a meta-analysis. PLoS One 2014;9:e111885. [Crossref] [PubMed]
- Wang Z, Sheng YY, Gao XM, et al. β-catenin mutation is correlated with a favorable prognosis in patients with hepatocellular carcinoma. Mol Clin Oncol 2015;3:936-40. [Crossref] [PubMed]
- Pinyol R, Sia D, Llovet JM. Immune Exclusion-Wnt/CTNNB1 Class Predicts Resistance to Immunotherapies in HCC. Clin Cancer Res 2019;25:2021-3. [Crossref] [PubMed]
- Ruiz de Galarreta M, Bresnahan E, Molina-Sánchez P, et al. β-Catenin Activation Promotes Immune Escape and Resistance to Anti-PD-1 Therapy in Hepatocellular Carcinoma. Cancer Discov 2019;9:1124-41. [Crossref] [PubMed]
- Chen L, Zhou Q, Liu J, et al. CTNNB1 Alternation Is a Potential Biomarker for Immunotherapy Prognosis in Patients With Hepatocellular Carcinoma. Front Immunol 2021;12:759565. [Crossref] [PubMed]
- Harding JJ, Nandakumar S, Armenia J, et al. Prospective Genotyping of Hepatocellular Carcinoma: Clinical Implications of Next-Generation Sequencing for Matching Patients to Targeted and Immune Therapies. Clin Cancer Res 2019;25:2116-26. [Crossref] [PubMed]
- Kuwano A, Yada M, Narutomi F, et al. Therapeutic efficacy of atezolizumab plus bevacizumab for hepatocellular carcinoma with WNT/β-catenin signal activation. Oncol Lett 2022;24:216. [Crossref] [PubMed]
- Tsujikawa H, Masugi Y, Yamazaki K, et al. Immunohistochemical molecular analysis indicates hepatocellular carcinoma subgroups that reflect tumor aggressiveness. Hum Pathol 2016;50:24-33. [Crossref] [PubMed]
- Dal Bello B, Rosa L, Campanini N, et al. Glutamine synthetase immunostaining correlates with pathologic features of hepatocellular carcinoma and better survival after radiofrequency thermal ablation. Clin Cancer Res 2010;16:2157-66. [Crossref] [PubMed]
- Loeppen S, Schneider D, Gaunitz F, et al. Overexpression of glutamine synthetase is associated with beta-catenin-mutations in mouse liver tumors during promotion of hepatocarcinogenesis by phenobarbital. Cancer Res 2002;62:5685-8. [PubMed]
- Calderaro J, Couchy G, Imbeaud S, et al. Histological subtypes of hepatocellular carcinoma are related to gene mutations and molecular tumour classification. J Hepatol 2017;67:727-38. [Crossref] [PubMed]
- Austinat M, Dunsch R, Wittekind C, et al. Correlation between beta-catenin mutations and expression of Wnt-signaling target genes in hepatocellular carcinoma. Mol Cancer 2008;7:21. [Crossref] [PubMed]
- Amato M, Franco R, Facchini G, et al. Microsatellite Instability: From the Implementation of the Detection to a Prognostic and Predictive Role in Cancers. Int J Mol Sci 2022;23:8726. [Crossref] [PubMed]
- Roudko V, Cimen Bozkus C, Greenbaum B, et al. Lynch Syndrome and MSI-H Cancers: From Mechanisms to "Off-The-Shelf" Cancer Vaccines. Front Immunol 2021;12:757804. [Crossref] [PubMed]
- Kawakami H, Zaanan A, Sinicrope FA. Microsatellite instability testing and its role in the management of colorectal cancer. Curr Treat Options Oncol 2015;16:30. [Crossref] [PubMed]
- Le DT, Uram JN, Wang H, et al. PD-1 Blockade in Tumors with Mismatch-Repair Deficiency. N Engl J Med 2015;372:2509-20. [Crossref] [PubMed]
- Marcus L, Lemery SJ, Keegan P, et al. FDA Approval Summary: Pembrolizumab for the Treatment of Microsatellite Instability-High Solid Tumors. Clin Cancer Res 2019;25:3753-8. [Crossref] [PubMed]
- Le DT, Durham JN, Smith KN, et al. Mismatch repair deficiency predicts response of solid tumors to PD-1 blockade. Science 2017;357:409-13. [Crossref] [PubMed]
- Kawaoka T, Ando Y, Yamauchi M, et al. Incidence of microsatellite instability-high hepatocellular carcinoma among Japanese patients and response to pembrolizumab. Hepatol Res 2020;50:885-8. [Crossref] [PubMed]
- Bartley AN, Mills AM, Konnick E, et al. Mismatch Repair and Microsatellite Instability Testing for Immune Checkpoint Inhibitor Therapy: Guideline From the College of American Pathologists in Collaboration With the Association for Molecular Pathology and Fight Colorectal Cancer. Arch Pathol Lab Med 2022;146:1194-210. [Crossref] [PubMed]
- Boland CR, Thibodeau SN, Hamilton SR, et al. A National Cancer Institute Workshop on Microsatellite Instability for cancer detection and familial predisposition: development of international criteria for the determination of microsatellite instability in colorectal cancer. Cancer Res 1998;58:5248-57. [PubMed]
- Samstein RM, Lee CH, Shoushtari AN, et al. Tumor mutational load predicts survival after immunotherapy across multiple cancer types. Nat Genet 2019;51:202-6. [Crossref] [PubMed]
- Jardim DL, Goodman A, de Melo Gagliato D, et al. The Challenges of Tumor Mutational Burden as an Immunotherapy Biomarker. Cancer Cell 2021;39:154-73. [Crossref] [PubMed]
- Newell F, Pires da Silva I, Johansson PA, et al. Multiomic profiling of checkpoint inhibitor-treated melanoma: Identifying predictors of response and resistance, and markers of biological discordance. Cancer Cell 2022;40:88-102.e7. [Crossref] [PubMed]
- Anagnostou V, Smith KN, Forde PM, et al. Evolution of Neoantigen Landscape during Immune Checkpoint Blockade in Non-Small Cell Lung Cancer. Cancer Discov 2017;7:264-76. [Crossref] [PubMed]
- Zhu J, Armstrong AJ, Friedlander TW, et al. Biomarkers of immunotherapy in urothelial and renal cell carcinoma: PD-L1, tumor mutational burden, and beyond. J Immunother Cancer 2018;6:4. [Crossref] [PubMed]
- Zhong C, Li Y, Yang J, et al. Immunotherapy for Hepatocellular Carcinoma: Current Limits and Prospects. Front Oncol 2021;11:589680. [Crossref] [PubMed]
- Cai H, Zhang Y, Zhang H, et al. Prognostic role of tumor mutation burden in hepatocellular carcinoma after radical hepatectomy. J Surg Oncol 2020;121:1007-14. [Crossref] [PubMed]
- Liu S, Tang Q, Huang J, et al. Prognostic analysis of tumor mutation burden and immune infiltration in hepatocellular carcinoma based on TCGA data. Aging (Albany NY) 2021;13:11257-80. [Crossref] [PubMed]
- Liu T, Tan J, Wu M, et al. High-affinity neoantigens correlate with better prognosis and trigger potent antihepatocellular carcinoma (HCC) activity by activating CD39(+)CD8(+) T cells. Gut 2021;70:1965-77. [Crossref] [PubMed]
- Mauriello A, Zeuli R, Cavalluzzo B, et al. High Somatic Mutation and Neoantigen Burden Do Not Correlate with Decreased Progression-Free Survival in HCC Patients not Undergoing Immunotherapy. Cancers (Basel) 2019;11:1824. [Crossref] [PubMed]
- Marabelle A, Fakih M, Lopez J, et al. Association of tumour mutational burden with outcomes in patients with advanced solid tumours treated with pembrolizumab: prospective biomarker analysis of the multicohort, open-label, phase 2 KEYNOTE-158 study. Lancet Oncol 2020;21:1353-65. [Crossref] [PubMed]
- Hsiehchen D, Espinoza M, Valero C, et al. Impact of tumor mutational burden on checkpoint inhibitor drug eligibility and outcomes across racial groups. J Immunother Cancer 2021;9:e003683. [Crossref] [PubMed]
- Havel JJ, Chowell D, Chan TA. The evolving landscape of biomarkers for checkpoint inhibitor immunotherapy. Nat Rev Cancer 2019;19:133-50. [Crossref] [PubMed]
- De Lorenzo S, Tovoli F, Trevisani F. Mechanisms of Primary and Acquired Resistance to Immune Checkpoint Inhibitors in Patients with Hepatocellular Carcinoma. Cancers (Basel) 2022;14:4616. [Crossref] [PubMed]
- Benci JL, Xu B, Qiu Y, et al. Tumor Interferon Signaling Regulates a Multigenic Resistance Program to Immune Checkpoint Blockade. Cell 2016;167:1540-1554.e12. [Crossref] [PubMed]
- Shin DS, Zaretsky JM, Escuin-Ordinas H, et al. Primary Resistance to PD-1 Blockade Mediated by JAK1/2 Mutations. Cancer Discov 2017;7:188-201. [Crossref] [PubMed]
- Jung KH, Yoo W, Stevenson HL, et al. Multifunctional Effects of a Small-Molecule STAT3 Inhibitor on NASH and Hepatocellular Carcinoma in Mice. Clin Cancer Res 2017;23:5537-46. [Crossref] [PubMed]
- Tsimberidou AM, de Achaval S, Alibhai I, et al. First-in-man phase I clinical trial evaluating TTI-101, an orally bioavailable, small molecule inhibitor of STAT3, in patients with advanced solid tumors. J Clin Oncol 2021;39:TPS3158. [Crossref]
- Chen J, Gingold JA, Su X. Immunomodulatory TGF-β Signaling in Hepatocellular Carcinoma. Trends Mol Med 2019;25:1010-23. [Crossref] [PubMed]
- Park BV, Freeman ZT, Ghasemzadeh A, et al. TGFβ1-Mediated SMAD3 Enhances PD-1 Expression on Antigen-Specific T Cells in Cancer. Cancer Discov 2016;6:1366-81. [Crossref] [PubMed]