Molecular pathology and protein markers for pancreatic cancer: relevance in staging, in adjuvant therapy, in determination of minimal residual disease, and follow-up
Introduction
Pancreatic ductal adenocarcinoma (PDAC) is an aggressive malignancy arising from the exocrine cells of the pancreas. It accounts for approximately 90% of all pancreatic malignancies (1). It is the tenth-most common cause of cancer, and third-leading cause of cancer deaths in the United States. It is projected to become the second-leading cause of cancer deaths by 2030 based on current trends, surpassing colorectal cancer deaths (2). In 2022, it is estimated that there will be 62,210 new cases of pancreatic cancer and an estimated 49,830 deaths (3). The current reported 5-year overall survival (OS) rate for PDAC is 11%, which increased only modestly over the last decade, despite new treatment strategies and understanding of the molecular mechanisms PDAC carcinogenesis (4). The poor prognosis of PDAC is attributed to advanced stage of disease at the time of presentation. Approximately 80% of patients with PDAC are diagnosed with locally-advanced or metastatic disease, rendering them ineligible or high-risk for surgical resection (5). Many cases of PDAC progress unchecked because early-stage disease is typically asymptomatic (6). Symptoms are often vague and non-specific, such as abdominal bloating and changes in bowel habits. Jaundice and pruritis are tumor location dependent, and tend to occur at later stages of the disease (7). Prior data demonstrate that time to progression from an early primary lesion to metastatic disease takes an average of 18.5 years, providing a wide window for earlier intervention (8,9). An understanding of the scope of this disease demonstrates both the need and potential to improve our tools for early diagnosis.
Circulating biomarkers are used in the field of oncology with varying benefit for diagnosis, prognostication, and assessment of response to therapy for various malignancies. Ideal biomarkers should inform us of the dynamics and progression of disease and help identify effective treatment strategies. During and after therapy, circulating biomarkers should help detect disease early recurrence. Carbohydrate antigen (CA) 19-9 is the most commonly used biomarker for PDAC (10). Other biomarkers remain in various stages of investigation and validation, and/or are prohibitively expensive for widespread use. Identification of circulating serum proteins as biomarkers for PDAC is attractive given the minimally-invasive nature of blood sampling and established methods of protein detection, such as enzyme-linked immunosorbent assay (ELISA). We reviewed existing and potential circulating protein biomarkers for detection and prognosis of PDAC (Figure 1, Table 1).
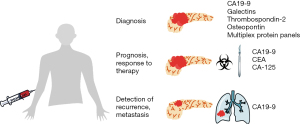
Table 1
Biomarker | Sensitivity (%) | Specificity (%) | Survival hazard ratio |
---|---|---|---|
Diagnostic | |||
CA19-9 (11) | 79–81 | 82–90 | – |
Galectins (12,13) | 64–96* | 71–100* | – |
Thrombospondin 2 (14-18) | 52–87* | 97–99* | – |
Osteopontin (19-21) | 34–87* | 91–94* | – |
Multiplex protein panels (22-24) | 76–92* | 78–92* | – |
Prognostic-predictive | |||
CA19-9 (10,25-37) | – | – | 1.26–2.0 |
CEA (38-43) | – | – | 1.1–2.23 |
CA-125 (44,45) | – | – | 1.5–2.1 |
Surveillance | |||
CA19-9 (46,47) | 78–90 | 62–83 | – |
Current and emerging protein biomarkers for PDAC are shown in the table. Pooled sensitivity and specificity ranges for diagnostic and surveillance biomarkers are as reported by references. Hazard ratio ranges are reported for prognostic-predictive biomarkers. *, denotes sensitivity or specificity measured when used in conjunction with CA19-9. CA, carbohydrate antigen; CEA, carcinoembryonic antigen; PDAC, pancreatic ductal adenocarcinoma.
Diagnostic protein biomarkers
CA19-9
CA19-9 is a sialylated CA form of a Lewis group antigen present on the surface of erythrocytes and other blood cells (48). It is commonly used to detect PDAC when clinical suspicion is high, based on clinical signs or imaging findings. In patients with symptoms such as jaundice, new-onset diabetes, unexplained weight loss, or upper abdominal or lumbar pain with suspected malignancy, the reported sensitivity and specificity of elevated CA19-9 to detect PDAC is 79–81% and 82–90%, respectively, using a cutoff value of >37 U/mL (11). While CA19-9 is measured when PDAC is suspected, some patients with a specific Lewis genotype (about 5–10% of the Caucasian population) are deemed “non-secretors” and do not express serum CA19-9, which typically provide false negative results (typically <1 U/mL) (49). Conversely, elevated CA19-9 levels may be associated with other malignant and non-malignant pathologies (Table 2). Patients with non-malignant obstructive jaundice may present with extreme elevation of CA19-9 that rapidly normalizes following relief of the obstruction. This is typically not observed in patients with obstructive jaundice caused by PDAC. These patients present with similarly elevated CA19-9 but CA19-9 levels remain elevated above normal after the obstruction is relieved (50).
Table 2
Benign | Malignant |
---|---|
Pancreatitis | Colorectal cancer |
Cirrhosis | Gastric cancer |
Acute cholangitis | Endometrial cancer |
Ascites | Cholangiocarcinoma |
Obstructive jaundice | Prostate cancer |
Systemic lupus erythematosus | Lung cancer |
Emphysema | Thyroid carcinoma |
Interstitial pneumonia | Transitional cell carcinoma |
Disorders associated with elevated serum CA19-9 are shown in the table. Elevations in serum CA19-9 may be biliary or non-biliary in origin. PDAC, pancreatic ductal adenocarcinoma; CA, carbohydrate antigen.
Given that elevation in CA19-9 is commonly observed in patients with PDAC, there was interest in using CA19-9 as a screening tool in healthy adults, and this was tested in a prospective study involving 70,940 asymptomatic individuals. PDAC was detected in only 4 patients among 1,063 individuals with elevated serum CA19-9 (0.3%), demonstrating that without other symptoms or risk factors, CA19-9 is not able to reliably identify PDAC (51).
Use of CA19-9 as a screening tool in high-risk populations, such as those with diabetes mellitus, chronic pancreatitis (CP), PDAC family history, and smoking, improves its diagnostic performance. The American College of Gastroenterology recommends that patients with high risk for PDAC (Table 3) should be screened with yearly endoscopic ultrasound alternating with magnetic resonance imaging (MRI) together with serum CA19-9 starting at the age of 50 years, or 10 years before the earliest age of a PDAC diagnosis in the family. These recommendations are based on evidence of increased risk of PDAC in these patient populations as compared with the general population, with the aim of earlier detection (52-63).
Table 3
Syndrome (reference) | Gene mutation (locus) | Relative risk of PDAC |
---|---|---|
Peutz-Jeghers syndrome (52) | STK11 (19q) | Up to 132-fold |
Hereditary pancreatitis (53) | PRSS1 (7q) | 53-fold |
Familial-atypical multiple mole melanoma (54) | CDKN2A (9p) | 13- to 39-fold |
Lynch syndrome (55) | MLH1 (3p), MSM2 (2p), MSM6 (2p), PMS2 (7p) | 9- to 11-fold |
Li-Fraumeni syndrome (56) | P53 (17p) | 7-fold |
Familial adenomatous polyposis (57) | APC (5q) | 5-fold |
Fanconi anemia (58) | FANCA (16q), FANCC (9q), FANCG (9p) | 4- to 6-fold |
Ataxia telangiectasia (59) | ATM (11q) | 3-fold |
Hereditary breast and ovarian cancer (60,61) | BRCA2 (13q), BRCA1 (13q), PALB2 (16p) | 2- to 9-fold |
The syndromes that are associated with increased risk of PDAC, the gene mutations responsible for these syndromes, and patients’ lifetime relative risk of PDAC compared to the general population are shown. The American College of Gastroenterology recommends screening for PDAC in individuals with these syndromes at regular intervals. PDAC, pancreatic ductal adenocarcinoma.
In summary, serum CA19-9 alone may be useful for screening or early detection of PDAC in high-risk populations when combined with endoscopic ultrasound and cross-sectional imaging, but it has limited value for these purposes in the general population. There may be additional value to screening the general populations with CA19-9 in conjunction with other circulating biomarkers, which will be discussed later in this review.
Galectins
Galectins are a family of proteins that bind to β-galactoside residues through a highly-conserved carbohydrate recognition domain, assisting in various biological functions including cell proliferation, transformation, adhesion, migration, and invasion (64). Intracellularly, cytosolic and nuclear galectins can regulate several cellular functions. For instance, they may regulate cellular growth and apoptosis through interaction with Ras and Bcl-2 molecules. Extracellularly, they may bind glycosylated membrane receptors and extracellular matrix proteins, modifying cell-cell interactions subsequently regulating immune and inflammatory responses (12,64). In the setting of PDAC, Gal-1, Gal-3, and Gal-9 have been studied for their role in oncogenesis and are under evaluation as potential biomarkers.
Gal-1 is a pro-tumoral lectin that increases cell proliferation, induces migration and invasion, enhances angiogenesis, permits immune evasion, and can induce acinar to ductal metaplasia (65). Gal-1 is not expressed in healthy pancreatic tissue, moderately-expressed in settings of pancreatitis, and most notably strongly-expressed in the stromal micro-environment surrounding the cancer mass, but not in the cancer cells themselves (66). A hallmark of PDAC is an abundant tumor stroma, accounting for up to 90% of tumor volume, and is proposed to be one of the main factors contributing to the aggressiveness of PDAC and its resistance to many therapies. Several studies support the role of Gal-1 expressed by activated stromal pancreatic stellate cells in promoting tumor size, perineural invasion, tumor stage, and differentiation through various autocrine signaling pathways (67-69). Stromal-derived Gal-1 also plays a role in paracrine signaling by modulating the immune microenvironment of pancreatic tumors and promoting immune evasion (66,70).
Considering the pivotal role that Gal-1 plays in PDAC progression, more recent studies sought to evaluate its efficacy as a diagnostic biomarker. A multicenter study by Martinez-Bosch et al. used serum samples from 90 patients with PDAC to evaluate the diagnostic efficacy of Gal-1 using ELISA for PDAC detection. They found a sensitivity of 75.0–77.4% and specificity of 71.4–100% at cutoff values of Gal-1 between 17.7–28.16 ng/mL when compared to healthy controls (13). When Gal-1 and CA19-9 were combined the sensitivity and specificity increased to 96% and 100%, respectively. This study demonstrates the utility of novel Gal-1 ELISA testing to complement CA19-9 in diagnosing PDAC.
Gal-3 is a pro-tumoral lectin localized to epithelial tumor cells and plays a similar role as Gal-1 through autocrine and paracrine signaling in tumor cell growth, migration, invasion, and immune escape. Notably, Gal-3 is not detected in normal pancreas but is overexpressed in around 85% of PDAC samples (71). A meta-analysis by Sun et al. established diagnostic value in the use of Gal-3. The pooled sensitivity of serum Gal-3 was 64% and specificity was 76%. Studies included in the meta-analysis proposed serum Gal-3 as a potential diagnostic biomarker for pancreatic cancer, and demonstrated that Gal-3, CA19-9, and CA-125 provided complementary diagnostic value for pancreatic cancer with a diagnostic sensitivity of 97.5% (12). However, other studies report mixed results when using Gal-3 as a diagnostic marker. A study by Coppin et al. argues that many previous studies failed to account for patients with pancreatic or liver fibrosis despite the well-studied role of galectins in inflammation and collagen production. The study demonstrates Gal-3 levels are similarly elevated in PDAC as well as in non-malignant pancreatic diseases, such as CP. The authors conclude that measuring Gal-3 provides little diagnostic value as it does not effectively discriminate PDAC versus other non-malignant pancreatic diseases (72). A study by Jiang et al. demonstrated FNA and cell-staining for Gal-3 was able to differentiate PDAC from non-neoplastic pancreatic tissue with a sensitivity of 95.7% and specificity of 87.5%. The study demonstrates that Gal-3 is uniquely and highly expressed in specimens of PDAC, but not pancreatic neuroendocrine neoplasm (PNEN) and gastrointestinal stromal tumors (GIST), proposing a role for Gal-3 in differentiating PDAC from other tumors such as PNEN and GIST. While preliminary evidence supports Gal-3 as a potential diagnostic biomarker, its usefulness in practical clinical application requires further study (73).
Gal-9 is a pro-tumoral protein localized to tumor epithelial cells that has been linked to immune reprogramming and evasion (74,75). The ligation of Gal-9 by dectin-1, an innate immune receptor expressed on the surface of macrophages, can drive macrophages into pro-tumorigenic and immunosuppressive M2 phenotype (76). A study by Seifert et al. including 70 PDAC, 18 CP, 18 intraductal papillary mucinous neoplasm (IPMN), and 28 healthy-individuals found that serum Gal-9 levels did not differ between healthy patients (median 6.68 ng/mL) and those with benign pancreatic disease (CP, median 6.44 ng/mL; IPMN, median 7.53 ng/mL), but subjects with PDAC had significantly higher serum Gal-9 (median 9.13 ng/mL). Notably, Gal-9 did not yield higher accuracy than CA19-9, but outperformed carcinoembryonic antigen (CEA) for the discrimination from benign pancreatic disease (77).
Thrombospondin-2 (THBS2)
THBS2 is a glycoprotein from the greater thrombospondin family that mediates cell-to-cell and cell-to-matrix interactions during tissue genesis and remodeling (78). Thrombospondins have important functions in immune responses, inflammation, cancer, growth, and development (79). Previous work suggests that THBS2 may promote cancer progression by remodeling the tumor microenvironment and enhancing matrix metalloproteinase expression (80). Kim et al. developed an induced pluripotent stem cell model from human PDAC cells that generated pancreatic intraepithelial neoplasia (PanIN) precursors that progressed to an invasive stage (81). They found that these PanIN organoids secreted proteins that fell into linked pathways for transforming growth factor beta (TGF-β) and integrin signaling. One such protein was THBS2, which is detectable via serum ELISA, making it an attractive potential diagnostic marker.
In another study, Kim and colleagues assessed the diagnostic role of THBS2 for PDAC utilizing patient samples from those with early- and late-stage PDAC, benign pancreatic diseases, and healthy subjects. Elevated THBS2 differentiated PDAC (n=197) from healthy subjects (n=140) with an area under the receiver operating characteristics curve (AUC) of 0.875. When THBS2 and CA19-9 were combined, the AUC increased to 0.970, indicating improved ability of the two biomarkers to differentiate PDAC from healthy controls. They then analyzed patients with early- and advanced-stage PDAC and found that THBS2 and CA19-9 together identified patients with resectable PDAC with an AUC of 0.960 and patients with locally-advanced or metastatic PDAC with an AUC of 0.980 when compared to healthy controls. The same authors performed an independent phase 2b validation study with an increased sample size of 537 patients with PDAC. They determined that using 42 ng/mL and 55 U/mL cutoff points for THBS2 and CA19-9, respectively, resulted in a specificity of 98% and a sensitivity of 87% to detect PDAC compared to healthy controls. The combination panel differentiated PDAC from IPMN with an AUC of 0.952 and PDAC from CP with an AUC of 0.867, while CA19-9 or THBS2 alone were not able to reliably differentiate patients with PDAC from those with IPMN or CP (14). A panel of THBS2 and CA19-9 may enable the early-stage detection of resectable PDAC while still maintaining the ability to distinguish PDAC from benign pancreatic disease.
Multiple other studies have redemonstrated the potential role of THBS2 ELISA in conjunction with CA19-9 to detect and diagnose PDAC (15-17). Simpson et al. extended this analysis and measured circulating levels of THBS2 in 164 patients with IPMN and to determine if changes in THBS2 elevation correlated to surgical dysplasia grade. Circulating THBS2 was greater in high-grade IPMN (26.6 ng/mL) than in low/moderate-grade IPMN (20.4 ng/mL), indicating that THBS2 may be useful as a marker to identify patients with high-grade dysplasia during normal IPMN surveillance (18).
Osteopontin (OPN)
OPN is a secreted phosphorylated glycoprotein that was originally isolated from bone and is an important factor in bone remodeling, anchoring osteoclasts to the mineral matrix of bones (82). However, its distribution is wider and it is upregulated at sites of inflammation and tissue remodeling (83). Recent studies demonstrated that OPN plays a role in numerous pathways that allow for tumor progression and metastasis in multiple solid tumors, including esophageal, gastric, liver, and pancreas cancers (84-87). Initial work demonstrated that OPN mRNA was increased in PDAC and CP tissues compared to normal pancreatic tissue, and immunohistochemical analysis found OPN staining in 60% of primary PDAC and 72% of lymph node and liver metastases (88). The same study measured circulating OPN levels via ELISA in PDAC (n=70), CP (n=12), and healthy donors (n=20) and found that serum OPN was increased 1.6-fold and 1.9-fold over healthy subjects, respectively. These authors concluded that while serum OPN was increased in PDAC, its use as a diagnostic marker may be limited given that serum levels were similar in CP. This study, however, was limited by CP sample size.
Subsequent studies have confirmed elevated serum OPN levels in PDAC but also demonstrated the ability to discriminate PDAC from CP using serum OPN, in contrast to prior findings (19-21). Poruk et al. analyzed serum OPN levels of patients with PDAC (n=86), CP (n=48), and healthy controls (n=86). They found a mean circulating OPN level of 77.6 ng/mL in patients with PDAC, which was significantly elevated compared to patients with CP (mean OPN 41.8 ng/mL) or healthy controls (mean OPN 39.5 mg/mL, P<0.0001). Serum OPN was not significantly different in patients with CP compared to healthy controls (20). Further analysis revealed that patients with resectable PDAC had significant elevation in serum OPN compared to CP and healthy controls (mean OPN 62.4 ng/mL, P=0.01), indicating the utility of OPN as an early diagnostic marker for PDAC. Another study examined OPN levels in PDAC (n=64), CP (n=71), type-II diabetes mellitus (T2DM, n=67), and healthy controls (n=48) and produced similar results, noting that circulating OPN was significantly higher in patients with PDAC compared to all other groups (P<0.001). Additionally, they found that patients with metastatic PDAC had significantly elevated OPN compared to patients with stage II or III disease. These authors proposed a cutoff level of 102 ng/mL OPN in conjunction with elevated serum levels of CA19-9 above 29 U/mL and found that AUC reached 0.88 with a sensitivity of 83% and specificity of 89% to discriminate patients with PDAC compared to CP, healthy controls, and T2DM (21).
Multiplex protein panels
Given the limited ability of single circulating biomarkers to identify early-stage PDAC, there is increasing interest in the use of multiplex protein biomarker panels. Multiple groups have described the use of such panels and have demonstrated superiority over CA19-9 alone in the diagnosis of early PDAC.
In a cohort study, a biomarker panel consisting of CA19-9, leucine-rich alpha-2-glycoprotein 1 (LRG1), and transthyretin (TTR) was developed with mass spectrometry and ELISA (22). LRG1 is a protein that directly binds to the TGF-β accessory receptor and promotes the pro-angiogenic Smad1/5/8 signaling pathway (89). Elevated levels of LRG1 have been found in the blood of patients with non-small cell lung cancer, colorectal cancer, and PDAC (90). TTR is a functional protein found in the pancreatic β-cell and is involved in promoting insulin release and protecting against β-cell death. TTR can enter the pancreatic duct system due to hyperplasia and architectural destruction in PDAC tissues (91). Researchers found that levels of CA19-9 and LRG1 increased, while levels of TTR declined in patients with PDAC compared to healthy controls. The three-marker panel had a sensitivity and specificity of 82.5% and 92.1%, respectively, in distinguishing PDAC patients (n=80) from healthy subjects (n=89). The use of this panel for the early detection of PDAC was assessed using sera from patients with stage I or II PDAC (n=50) compared to healthy subjects (n=89). CA19-9 had an AUC value of 0.792 (sensitivity =64.0%), whereas the combined biomarkers improved the AUC value to 0.907–0.914 with a sensitivity of 76.0% and a specificity of 78.0%. Compared to CA19-9 alone (AUC =0.812), the panel of CA19-9, LRG1, and TTR discriminated PDAC from IPMN with an AUC value of 0.895 (specificity =85.7%; sensitivity =82.5%) (22). This retrospective study demonstrated the panel’s high diagnostic accuracy and ability to complement CA19-9 in detecting early-stage PDAC.
A panel consisting of TFF1, TFF2, and TFF3 (trefoil factors 1, 2, and 3, respectively) was evaluated in 80 patients with early-stage PDAC (I and II), 47 patients with CP, and 104 healthy controls (23). TFFs are stable mucin-associated proteins expressed in the gastrointestinal mucosa. They protect the gastric mucosa from inflammation and accelerate epithelial healing after injury (92). Their role as oncogenes was observed in multiple malignancies, including colon, breast, prostate, and ovarian cancers (93). A combination of all TFFs and CA19-9 had a sensitivity of 85% and a specificity of 92% for the detection of stages I and II PDAC compared to healthy controls with an AUC of 0.93. This panel also was superior over CA19-9 alone in its ability to differentiate CP from early-stage PDAC, with a sensitivity and specificity of 92% and an AUC of 0.94 (23).
Buoyed by results indicating that the use of biomarkers in conjunction with CA19-9 improves the detection of PDAC, Song et al. used magnetic bead sorting to develop an immunoassay of six circulating biomarkers. The use of magnetic beads improves throughput and allows for the measurement of multiple proteins from one sample. They chose to measure macrophage inhibitory cytokine-1 (MIC-1), carcinoembryonic antigen cell adhesion molecule-1 (CEACAM-1), OPN, melanoma inhibitory activity (MIA), spondin-1 (SPON1), and heat shock protein 27 (HSP27) to attempt to detect early PDAC compared to CA19-9 alone (24). These biomarkers were chosen based on previous research implicating their role in the pathogenesis of PDAC and prior studies demonstrating elevation in the sera of patients with PDAC (19,21,86,94-96). This panel was evaluated in patients with PDAC (n=188), IPMN (n=63), CP (n=68), and healthy controls (n=89). They found that SPON1 and HSP27 were not able to discriminate PDAC from healthy controls and were not included in their final analysis. They found that a two-marker panel of CA19-9 and MIA significantly improved the differentiation of early-stage PDAC from CP with an AUC of 0.86. In addition, they reported that CA19-9 and MIC-1 together could distinguish early-stage PDAC from IPMN with an AUC of 0.81. A panel consisting of CA19-9, MIC-1, and OPN was more successful than the individual biomarkers in the differentiation of IPMN from CP, with an AUC of 0.81. A three-marker panel of CA19-9, CEACAM-1, and MIA differentiated patients with PDAC from CP, with an AUC of 0.86. These results demonstrate the feasibility of multiplex assay technology in the diagnosis of PDAC (24).
Accumulating data show that combinations of biomarkers are more effective and accurate than single biomarkers in the diagnosis of PDAC. Serum biomarker panels reach sensitivity, specificity, and overall accuracy levels unlikely for a single biomarker such as CA19-9. The combination of markers might improve early diagnosis of patients with PDAC. However, these studies are limited by small sample sizes and require more investigation.
Prognostic protein biomarkers
CA19-9
Previous studies demonstrate that CA19-9 is limited in its utility to diagnose PDAC, but several studies show that changes in CA19-9 can predict response to neoadjuvant therapy and overall prognosis. Authors of a large retrospective study examined correlations between CA19-9 levels and prognosis in patients diagnosed with PDAC. They found that patients with normal as compared to increased CA19-9 levels had an associated hazard ratio (HR) for death between 0.68 (advanced stages III–IV) and 0.77 (stages I–II) (97). Results from another study of 152 patients with unresectable PDAC showed that OS and progression-free survival (PFS) were shorter in PDAC patients with CA19-9 ≥90 U/mL than in a group with a CA19-9 of <90 U/mL (PFS 4.4 vs. 17.0 months, respectively, P<0.001; OS 7.4 vs. 26.1 months, respectively, P<0.001) (98).
In patients with localized PDAC treated with a surgery-first approach, multiple investigators demonstrated that preoperative CA19-9 levels correlate with tumor stage, resectability, and risk of recurrence and survival (25-29). Ferrone et al. examined 176 patients with localized PDAC and found that CA19-9 correlated with the American Joint Committee on Cancer (AJCC) pathological stage, as well as post-resection survival. Patients with preoperative CA19-9 values greater than 1,000 U/mL had a median OS of only 12 months compared to 28 months for patients with CA19-9 values <1,000 U/mL (25). Hartwig et al. observed a strong inverse relationship between preoperative CA19-9 levels and both R0 resection rates and OS. In their study, 312 patients had a pre-treatment CA19-9 level >1,000 U/mL and in this subgroup, there were no 5-year survivors and the median OS after resection was approximately 12 months. As a result, the authors concluded that patients with CA19-9 levels >1,000 are at a high risk for the development of recurrence or metastatic disease and a neoadjuvant treatment approach should be considered (27). Bergquist et al. demonstrated in their analysis of patients in the National Cancer Database (NCDB) that elevation of CA19-9 is associated with decreased stage-specific survival, with the greatest difference in early stages. They suggest that patients who present with elevated CA19-9 levels at diagnosis have biologically borderline resectable pathology, regardless of anatomic resectability on imaging and recommend these patients undergo neoadjuvant chemotherapy (NAC) prior to surgical intervention (10). This data is bolstered by similar studies showing that CA19-9 may be prognostic of resectability in patients with PDAC. It has been reported that patients with a median CA19-9 level of <130 U/mL are more likely to have resectable tumors than patients with higher levels (30).
Work by Aldakkak et al. suggests that CA19-9 levels at initial presentation are not entirely predictive of how a patient will respond to NAC (31). Instead, researchers postulate that CA19-9 kinetics, rather than overall level can be useful for measuring the response after NAC and predict outcomes should a patient undergo surgery. A multicenter study included 274 PDAC patients who received NAC with 5-fluorouracil, folinic acid, oxaliplatin, and irinotecan (FOLFIRINOX) (FLX) or gemcitabine/nab-paclitaxel (GNP) followed by curative-intentioned pancreatectomy. The authors analyzed the clinical and biochemical response to NAC. The results showed that a biochemical response characterized by decrease in the overall level of CA19-9 by greater than 50% was associated with better OS, recurrence-free survival (RFS), and metastasis-free survival (MFS) rates compared to patients whose CA19-9 decreased by less than 50% after NAC (OS: 42.3 vs. 24.3 months, P<0.001; RFS: 27.3 vs. 14.1 months, P=0.042; MFS: 29.3 vs. 13 months, P=0.047). This study suggests that a biochemical response after NAC is predictive of increased survival (32). Recently, the results of a retrospective analysis regarding the usefulness of multiple assessments of CA19-9 levels after NAC and subsequent resection were published. It was concluded that a CA19-9 level of ≤103 U/mL, tumor size of ≤27 mm, and the lack of lymph node metastasis and R0 resection were significant predictors of survival benefits. Moreover, PDAC patients with elevated CA19-9 levels of >37 U/mL before NAC with normalization of CA19-9 to ≤37 U/mL after NAC were associated with a lower risk of hepatic recurrence (18%) compared to patients with CA19-9 >37 U/mL after NAC (31%). The authors suggested that CA19-9 monitoring could be used to assess the efficiency of NAC therapy (33).
Current guidelines recommend that patients with borderline resectable (BRPC) and locally advanced pancreatic cancer (LAPC) undergo NAC prior to evaluation for surgical resection (34). The decision about which patients are qualified for potential resection following NAC is clinically difficult because 40% or more of patients with BRPC or LAPC who receive NAC do not reach resection (35). Heger et al. investigated CA19-9 levels of 318 patients during NAC to predict tumor resectability. CA19-9 levels were analyzed at diagnosis and before and after FLX or GNP NAC in patients with BRPC and LAPC. The study showed that a decrease in the CA19-9 concentration to a value lower than 91.8 U/mL after FLX NAC could independently predict tumor resectability in patients with BRPC or LAPC and correlated to a median OS of 23 months. For patients with a post-NAC CA19-9 level above 91.8 U/mL, resection did not improve median OS compared to patients who underwent exploration only. The authors suggested that a decrease in CA19-9 levels after NAC may predict tumor resectability and should be included as one of the qualifying factors for surgical decision making (36). In patients with unresectable disease, Yang et al. measured CA19-9 levels before and after chemoradiation therapy (CRT). Following CRT, patients with a decrease of >90% from their baseline CA19-9 level had a significantly improved median survival than those that did not (16.2 vs. 7.5 months, P=0.01). The median survival of patients with a CA19-9 level lower than the median post CRT value was 10.3 months, compared with 7.1 months for those with a CA19-9 level greater than the median (P=0.03) (37).
Altogether, numerous studies have demonstrated that CA19-9 level at diagnosis can not only provide prognostic information, but changes in CA19-9 can predict response to therapy. These studies are limited in that most are retrospective in nature. Additionally, a standard cutoff value has not been implemented, making comparison across studies difficult. More prospective data is necessary to establish widely-accepted guidelines regarding the use of CA19-9 as a predictive biomarker.
CEA
CEA is a surface glycoprotein that facilitates cell adhesion. Normally produced by the fetal gastrointestinal tract, its production is halted before birth, with postnatal levels declining to below 20 ng/mL in adults (99). It is commonly used in the diagnosis of colon cancer, and it is elevated in up to 60% of patients with PDAC (100). CEA is limited as a diagnostic marker compared to CA19-9 because while it is equally specific, it is less sensitive in detecting PDAC (101). CEA can be a useful biomarker for prognostication as well as a predictor of early recurrence after surgical intervention. A retrospective analysis by Lee et al. (n=187) demonstrated that patents with elevated CEA at diagnosis had larger tumors and were more likely to present with advanced stage disease (38). They also found that the median OS of the normal serum CEA group was longer than that of the elevated serum CEA group (16.3 vs. 10.2 months, P=0.004) when a cutoff value of 5 ng/mL was used, and was independently associated with poor survival on multivariable analysis, regardless of tumor resectability (HR: 1.61, 95% CI: 1.07–2.42, P=0.022). These findings have been reconfirmed in multiple studies, with CEA elevation independently associated with poor survival following resection in patients who underwent upfront surgical resection or received neoadjuvant CRT (39-42). Recently, in a retrospective review of 319 patients with localized PDAC, Kato et al. found that high CEA level pre-neoadjuvant CRT was the most significant independent predictor of poor post-surgical disease-free survival (DFS) and OS (43).
CA-125
CA-125, also known as mucin-16 (MUC-16) is a mucin glycoprotein that is widely expressed at cell surfaces and plays a role in the barrier immunity of mucosal surfaces (99). It primarily known as a useful serological marker for the clinical management of ovarian cancer (102). It is a commonly measured tumor marker during initial diagnosis of PDAC, however its value in diagnosing PDAC has been limited (103). Emerging data suggest that CA-125 plays a role in the metastasis of pancreatic neoplasms and preoperative elevation in CA-125 may predict the presence of metastatic disease that is undetectable by computed tomography (CT) (44). One study retrospectively analyzed the records of 905 patients and collected prospective data from 142 patients with PDAC and found that serum CA-125 levels were the most strongly associated with metastasis. Patients with distant metastatic disease had an average serum CA-125 level of 72.3 U/mL, compared to patients with unresectable LAPC (27.0 U/mL) and resectable PDAC (13.3 U/mL). In patients undergoing resection, those with greater than three positive lymph nodes on pathology had significantly higher baseline CA-125 (30.1 U/mL) than those 1–3 positive lymph nodes (18.7 U/mL) or no positive lymph nodes (13.1 U/mL). High baseline CA-125 levels above a cutoff value of 18.4 U/mL predicted early distant metastasis within after pancreatectomy compared to patients below the cutoff (55/165 vs. 6/108, P<0.001). These patients with elevated baseline CA-125 also had shorter median OS (11.3 vs. 25.3 months, P<0.001) and RFS (6.1 vs. 17.6 months, P<0.001) after resection (45). These data suggest that CA-125 can be used as a predictor of metastasis, however these studies were conducted solely in an Asian population. More studies are necessary to examine the predictive value of CA-125 in an expanded population.
Surveillance protein biomarkers
CA19-9
Following pancreatectomy for PDAC, close surveillance is necessary to detect recurrence because at least 80% of patients will develop local and/or distant disease recurrence, often within two years (104,105). Without recurrence-focused surveillance, disease recurrence may not be identified until after the manifestation of symptoms, at which point recurrence is usually advanced and is associated with worse prognosis (106). The National Comprehensive Cancer Network (NCCN) guidelines recommend surveillance every 3–6 months for two years and then every 6–12 months as indicated with clinic visit, cross sectional imaging of the chest, abdomen, and pelvis, as well as measurement of CA19-9 levels (34). CA19-9 can be used to detect tumor recurrence and predict long-term survival. Surveillance imaging may not be sufficient to detect small or diffuse foci of metastasis located in the peritoneum, which might lead to delays in starting relevant treatment (36).
Rieser et al. observed CA19-9 levels after pancreatectomy and found that CA19-9 predicts RFS and may help direct protocols of salvage chemotherapy. The study included 525 patients undergoing surgery for PDAC. The authors identified different patterns of CA19-9 behavior at baseline and after resection. Patients whose CA19-9 remained persistently high from diagnosis through treatment had significantly worse RFS and OS, while CA19-9 normalization following treatment was an independent predictor of RFS and OS. Additionally, the authors compared the increase of CA19-9 at 6-month intervals and radiographic findings. The results showed that for radiographic recurrence, elevated levels of CA19-9 had poor positive predictive value (average: 35%) but the normalization of CA19-9 had high negative predictive value (average: 92%). The authors posited that normal CA19-9 levels indicate no or very low risk of recurrence on imaging, but elevated CA19-9 levels are frequently discordant with recurrence seen on CT, and that a rise in CA19-9 may precede radiologically significant recurrence by 6 months (46).
The connection between increases in CA19-9 and radiological recurrence was investigated in a follow-up study after PDAC resection. CA19-9 was measured at diagnosis, after surgery, after adjuvant chemotherapy, and at a validation point in 134 patients and compared against CT and MRI findings. The authors observed that approximately 60% of the patients after resection had significantly elevated CA19-9 values before the detection of recurrence in imaging techniques. Additionally, in the validation set, a CA19-9 level 2.45 times above normal was found to indicate recurrence with a sensitivity of 90% and a specificity of 83.33%, with an AUC of 0.95. Concomitant CA19-9 elevation and CT recurrence detection was found only in four patients. The authors suggested that CA19-9 monitoring after PDAC resection could be helpful in directing patient management even without imaging results. Close CA19-9 observation could detect PDAC recurrence or metastases, even several months before their clinical or radiologic evidence. Therefore, these data suggest that the routine monitoring of CA19-9 levels may improve patient outcomes and survival (47).
Elevated postoperative CA19-9 levels were found to be correlated with worse prognosis and hepatic recurrence after surgery. A recent study enrolled 539 consecutive patients with PDAC who underwent R0 resection and stratified them into two groups: patients with sustained high levels of CA19-9 after surgery and patients with no postoperative elevation of CA19-9. It was found that postoperative sustained elevation was associated with a shorter median OS compared to patients with normal levels of CA19-9 (17.1 vs. 35.4 months, respectively; P<0.0001). Postoperative CA19-9 elevation was 2.6 times more consistent with hepatic recurrence than in the group without elevation (45% vs. 17%, respectively; P<0.0001) (107). Postoperative CA19-9 elevation after resection is a strong independent predictor for survival and could indicate the presence of occult distant metastasis in patients with PDAC. Furthermore, these studies indicate that patients with increased postoperative CA19-9 levels may require intensive adjuvant therapy.
In summary, CA19-9 serum measurement is becoming more useful in assessing treatment efficacy and predicting outcome, recurrence, and response to chemotherapy than in the early diagnosis of PDAC. The routine monitoring of CA19-9 levels after NAC or surgical intervention could detect PDAC recurrence or metastases before their clinical or radiologic evidence.
Non-protein molecular biomarkers of pancreatic adenocarcinoma
Serum proteins are not the only class of circulating biomarker that is under exploration for use in patients with PDAC. Circulating tumor cells (CTCs) and circulating tumor DNA (ctDNA) exosomes not discussed have all emerged in recent years as tools to diagnose, predict response to therapies, or detect recurrence. CTCs are a rare subset of tumor cells that are found in the bloodstream of patients with solid tumors and are thought to be the “seeds” of metastasis (108). CTC isolation allows for in-depth characterization of solid tumor cells, including metabolomic and proteomic analysis, and potential to culture for personalized treatments (109). ctDNA is released into the bloodstream as unencapsulated or partially encapsulated nucleic acids when PDAC cells undergo apoptosis or necrosis (110). It can provide clinicians information regarding PDAC as it harbors mutations or epigenetic characteristics specific to the patient’s cancerous process (111). These new biomarkers have the potential to be combined with protein biomarkers like CA19-9 to improve detection or devise individualized approaches to therapy (112,113). These emerging technologies are still in the early investigative phase, with few large studies available, and robust prospective trials are required.
Conclusions
In recent years, many studies identifying novel PDAC biomarkers and biomarker panels have emerged. However, none of these biomarkers are currently established in clinical practice. These biomarker studies were based on relatively low case numbers and suffer from a lack of validation. With further investigation, these novel biomarkers and biomarker panels have the potential to increase our ability to detect early-stage PDAC in conjunction with CA19-9.
Further understanding the complexities of cancer biology can help develop clinically useful markers for the early detection and prognosis of PDAC. Large prospective studies are needed to investigate the clinical impact of including these biomarkers in clinical decision making to improve outcomes for this disease. The discovery of accurate biomarkers may also allow for the better stratification of patients and guide therapeutic choices.
Acknowledgments
Funding: None.
Footnote
Provenance and Peer Review: This article was commissioned by the editorial office, Hepatobiliary Surgery and Nutrition for the series “Molecular, Protein, and Cellular Markers for HPB Cancers”. The article has undergone external peer review.
Conflicts of Interest: All authors have completed the ICMJE uniform disclosure form (available at https://hbsn.amegroups.com/article/view/10.21037/hbsn-22-628/coif). The series “Molecular, Protein, and Cellular Markers for HPB Cancers” was commissioned by the editorial office without any funding or sponsorship. D.A.K. served as the unpaid Guest Editor of the series. The authors have no other conflicts of interest to declare.
Ethical Statement: The authors are accountable for all aspects of the work in ensuring that questions related to the accuracy or integrity of any part of the work are appropriately investigated and resolved.
Open Access Statement: This is an Open Access article distributed in accordance with the Creative Commons Attribution-NonCommercial-NoDerivs 4.0 International License (CC BY-NC-ND 4.0), which permits the non-commercial replication and distribution of the article with the strict proviso that no changes or edits are made and the original work is properly cited (including links to both the formal publication through the relevant DOI and the license). See: https://creativecommons.org/licenses/by-nc-nd/4.0/.
References
- Saad AM, Turk T, Al-Husseini MJ, et al. Trends in pancreatic adenocarcinoma incidence and mortality in the United States in the last four decades; a SEER-based study. BMC Cancer 2018;18:688. [Crossref] [PubMed]
- Rahib L, Wehner MR, Matrisian LM, et al. Estimated Projection of US Cancer Incidence and Death to 2040. JAMA Netw Open 2021;4:e214708. [Crossref] [PubMed]
- Siegel RL, Miller KD, Fuchs HE, et al. Cancer statistics, 2022. CA Cancer J Clin 2022;72:7-33. [Crossref] [PubMed]
- Conroy T, Hammel P, Hebbar M, et al. FOLFIRINOX or Gemcitabine as Adjuvant Therapy for Pancreatic Cancer. N Engl J Med 2018;379:2395-406. [Crossref] [PubMed]
- Rawla P, Sunkara T, Gaduputi V. Epidemiology of Pancreatic Cancer: Global Trends, Etiology and Risk Factors. World J Oncol 2019;10:10-27. [Crossref] [PubMed]
- Kapszewicz M, Małecka-Wojciesko E. Simple Serum Pancreatic Ductal Adenocarcinoma (PDAC) Protein Biomarkers-Is There Anything in Sight? J Clin Med 2021;10:5463. [Crossref] [PubMed]
- Elbanna KY, Jang HJ, Kim TK. Imaging diagnosis and staging of pancreatic ductal adenocarcinoma: a comprehensive review. Insights Imaging 2020;11:58. [Crossref] [PubMed]
- Yachida S, Iacobuzio-Donahue CA. Evolution and dynamics of pancreatic cancer progression. Oncogene 2013;32:5253-60. [Crossref] [PubMed]
- Yachida S, Jones S, Bozic I, et al. Distant metastasis occurs late during the genetic evolution of pancreatic cancer. Nature 2010;467:1114-7. [Crossref] [PubMed]
- Bergquist JR, Puig CA, Shubert CR, et al. Carbohydrate Antigen 19-9 Elevation in Anatomically Resectable, Early Stage Pancreatic Cancer Is Independently Associated with Decreased Overall Survival and an Indication for Neoadjuvant Therapy: A National Cancer Database Study. J Am Coll Surg 2016;223:52-65. [Crossref] [PubMed]
- Ballehaninna UK, Chamberlain RS. The clinical utility of serum CA 19-9 in the diagnosis, prognosis and management of pancreatic adenocarcinoma: An evidence based appraisal. J Gastrointest Oncol 2012;3:105-19. [Crossref] [PubMed]
- Sun Q, Zhang Y, Liu M, et al. Prognostic and diagnostic significance of galectins in pancreatic cancer: a systematic review and meta-analysis. Cancer Cell Int 2019;19:309. [Crossref] [PubMed]
- Martinez-Bosch N, Barranco LE, Orozco CA, et al. Increased plasma levels of galectin-1 in pancreatic cancer: potential use as biomarker. Oncotarget 2018;9:32984-96. [Crossref] [PubMed]
- Kim J, Bamlet WR, Oberg AL, et al. Detection of early pancreatic ductal adenocarcinoma with thrombospondin-2 and CA19-9 blood markers. Sci Transl Med 2017;9:eaah5583. [Crossref] [PubMed]
- Berger AW, Schwerdel D, Reinacher-Schick A, et al. A Blood-Based Multi Marker Assay Supports the Differential Diagnosis of Early-Stage Pancreatic Cancer. Theranostics 2019;9:1280-7. [Crossref] [PubMed]
- Peng HY, Chang MC, Hu CM, et al. Thrombospondin-2 is a Highly Specific Diagnostic Marker and is Associated with Prognosis in Pancreatic Cancer. Ann Surg Oncol 2019;26:807-14. [Crossref] [PubMed]
- Le Large TYS, Meijer LL, Paleckyte R, et al. Combined Expression of Plasma Thrombospondin-2 and CA19-9 for Diagnosis of Pancreatic Cancer and Distal Cholangiocarcinoma: A Proteome Approach. Oncologist 2020;25:e634-43. [Crossref] [PubMed]
- Simpson RE, Yip-Schneider MT, Wu H, et al. Circulating Thrombospondin-2 enhances prediction of malignant intraductal papillary mucinous neoplasm. Am J Surg 2019;217:425-8. [Crossref] [PubMed]
- Koopmann J, Rosenzweig CN, Zhang Z, et al. Serum markers in patients with resectable pancreatic adenocarcinoma: macrophage inhibitory cytokine 1 versus CA19-9. Clin Cancer Res 2006;12:442-6. [Crossref] [PubMed]
- Poruk KE, Firpo MA, Scaife CL, et al. Serum osteopontin and tissue inhibitor of metalloproteinase 1 as diagnostic and prognostic biomarkers for pancreatic adenocarcinoma. Pancreas 2013;42:193-7. [Crossref] [PubMed]
- Rychlíková J, Vecka M, Jáchymová M, et al. Osteopontin as a discriminating marker for pancreatic cancer and chronic pancreatitis. Cancer Biomark 2016;17:55-65. [Crossref] [PubMed]
- Park J, Choi Y, Namkung J, et al. Diagnostic performance enhancement of pancreatic cancer using proteomic multimarker panel. Oncotarget 2017;8:93117-30. [Crossref] [PubMed]
- Jahan R, Ganguly K, Smith LM, et al. Trefoil factor(s) and CA19.9: A promising panel for early detection of pancreatic cancer. EBioMedicine 2019;42:375-85. [Crossref] [PubMed]
- Song J, Sokoll LJ, Pasay JJ, et al. Identification of Serum Biomarker Panels for the Early Detection of Pancreatic Cancer. Cancer Epidemiol Biomarkers Prev 2019;28:174-82. [Crossref] [PubMed]
- Ferrone CR, Finkelstein DM, Thayer SP, et al. Perioperative CA19-9 levels can predict stage and survival in patients with resectable pancreatic adenocarcinoma. J Clin Oncol 2006;24:2897-902. [Crossref] [PubMed]
- Barton JG, Bois JP, Sarr MG, et al. Predictive and prognostic value of CA 19-9 in resected pancreatic adenocarcinoma. J Gastrointest Surg 2009;13:2050-8. [Crossref] [PubMed]
- Hartwig W, Strobel O, Hinz U, et al. CA19-9 in potentially resectable pancreatic cancer: perspective to adjust surgical and perioperative therapy. Ann Surg Oncol 2013;20:2188-96. [Crossref] [PubMed]
- Humphris JL, Chang DK, Johns AL, et al. The prognostic and predictive value of serum CA19.9 in pancreatic cancer. Ann Oncol 2012;23:1713-22. [Crossref] [PubMed]
- Turrini O, Schmidt CM, Moreno J, et al. Very high serum CA 19-9 levels: a contraindication to pancreaticoduodenectomy? J Gastrointest Surg 2009;13:1791-7. [Crossref] [PubMed]
- Maithel SK, Maloney S, Winston C, et al. Preoperative CA 19-9 and the yield of staging laparoscopy in patients with radiographically resectable pancreatic adenocarcinoma. Ann Surg Oncol 2008;15:3512-20. [Crossref] [PubMed]
- Aldakkak M, Christians KK, Krepline AN, et al. Pre-treatment carbohydrate antigen 19-9 does not predict the response to neoadjuvant therapy in patients with localized pancreatic cancer. HPB (Oxford) 2015;17:942-52. [Crossref] [PubMed]
- Macedo FI, Ryon E, Maithel SK, et al. Survival Outcomes Associated With Clinical and Pathological Response Following Neoadjuvant FOLFIRINOX or Gemcitabine/Nab-Paclitaxel Chemotherapy in Resected Pancreatic Cancer. Ann Surg 2019;270:400-13. [Crossref] [PubMed]
- Aoki S, Motoi F, Murakami Y, et al. Decreased serum carbohydrate antigen 19-9 levels after neoadjuvant therapy predict a better prognosis for patients with pancreatic adenocarcinoma: a multicenter case-control study of 240 patients. BMC Cancer 2019;19:252. [Crossref] [PubMed]
- Network NCC. Pancreatic Adenocarcinoma (Version: 1.2022) 2022. Available online: https://www.nccn.org/professionals/physician_gls/pdf/pancreatic.pdf. Accessed October 26, 2022.
- Hackert T, Sachsenmaier M, Hinz U, et al. Locally Advanced Pancreatic Cancer: Neoadjuvant Therapy With Folfirinox Results in Resectability in 60% of the Patients. Ann Surg 2016;264:457-63. [Crossref] [PubMed]
- Heger U, Sun H, Hinz U, et al. Induction chemotherapy in pancreatic cancer: CA 19-9 may predict resectability and survival. HPB (Oxford) 2020;22:224-32. [Crossref] [PubMed]
- Yang GY, Malik NK, Chandrasekhar R, et al. Change in CA 19-9 levels after chemoradiotherapy predicts survival in patients with locally advanced unresectable pancreatic cancer. J Gastrointest Oncol 2013;4:361-9. [Crossref] [PubMed]
- Lee KJ, Yi SW, Chung MJ, et al. Serum CA 19-9 and CEA levels as a prognostic factor in pancreatic adenocarcinoma. Yonsei Med J 2013;54:643-9. [Crossref] [PubMed]
- Wu L, Huang P, Wang F, et al. Relationship between serum CA19-9 and CEA levels and prognosis of pancreatic cancer. Ann Transl Med 2015;3:328. [Crossref] [PubMed]
- Distler M, Pilarsky E, Kersting S, et al. Preoperative CEA and CA 19-9 are prognostic markers for survival after curative resection for ductal adenocarcinoma of the pancreas - a retrospective tumor marker prognostic study. Int J Surg 2013;11:1067-72. [Crossref] [PubMed]
- Li X, Li S, Liu L, et al. Effect of Perioperative CEA and CA24-2 on Prognosis of Early Resectable Pancreatic Ductal Adenocarcinoma. J Cancer 2020;11:9-15. [Crossref] [PubMed]
- Suzuki S, Shimoda M, Shimazaki J, et al. Predictive Early Recurrence Factors of Preoperative Clinicophysiological Findings in Pancreatic Cancer. Eur Surg Res 2018;59:329-38. [Crossref] [PubMed]
- Kato H, Kishiwada M, Hayasaki A, et al. Role of Serum Carcinoma Embryonic Antigen (CEA) Level in Localized Pancreatic Adenocarcinoma: CEA Level Before Operation is a Significant Prognostic Indicator in Patients With Locally Advanced Pancreatic Cancer Treated With Neoadjuvant Therapy Followed by Surgical Resection: A Retrospective Analysis. Ann Surg 2022;275:e698-707. [Crossref] [PubMed]
- Luo G, Xiao Z, Long J, et al. CA125 is superior to CA19-9 in predicting the resectability of pancreatic cancer. J Gastrointest Surg 2013;17:2092-8. [Crossref] [PubMed]
- Liu L, Xu HX, Wang WQ, et al. Serum CA125 is a novel predictive marker for pancreatic cancer metastasis and correlates with the metastasis-associated burden. Oncotarget 2016;7:5943-56. [Crossref] [PubMed]
- Rieser CJ, Zenati M, Hamad A, et al. CA19-9 on Postoperative Surveillance in Pancreatic Ductal Adenocarcinoma: Predicting Recurrence and Changing Prognosis over Time. Ann Surg Oncol 2018;25:3483-91. [Crossref] [PubMed]
- Azizian A, Rühlmann F, Krause T, et al. CA19-9 for detecting recurrence of pancreatic cancer. Sci Rep 2020;10:1332. [Crossref] [PubMed]
- Martin LK, Wei L, Trolli E, et al. Elevated baseline CA19-9 levels correlate with adverse prognosis in patients with early- or advanced-stage pancreas cancer. Med Oncol 2012;29:3101-7. [Crossref] [PubMed]
- Parra-Robert M, Santos VM, Canis SM, et al. Relationship Between CA 19.9 and the Lewis Phenotype: Options to Improve Diagnostic Efficiency. Anticancer Res 2018;38:5883-8. [Crossref] [PubMed]
- Hata S, Sakamoto Y, Yamamoto Y, et al. Prognostic impact of postoperative serum CA 19-9 levels in patients with resectable pancreatic cancer. Ann Surg Oncol 2012;19:636-41. [Crossref] [PubMed]
- Kim JE, Lee KT, Lee JK, et al. Clinical usefulness of carbohydrate antigen 19-9 as a screening test for pancreatic cancer in an asymptomatic population. J Gastroenterol Hepatol 2004;19:182-6. [Crossref] [PubMed]
- Giardiello FM, Brensinger JD, Tersmette AC, et al. Very high risk of cancer in familial Peutz-Jeghers syndrome. Gastroenterology 2000;119:1447-53. [Crossref] [PubMed]
- Lowenfels AB, Maisonneuve P, DiMagno EP, et al. Hereditary pancreatitis and the risk of pancreatic cancer. International Hereditary Pancreatitis Study Group. J Natl Cancer Inst 1997;89:442-6. [Crossref] [PubMed]
- Vasen HF, Gruis NA, Frants RR, et al. Risk of developing pancreatic cancer in families with familial atypical multiple mole melanoma associated with a specific 19 deletion of p16 (p16-Leiden). Int J Cancer 2000;87:809-11.
- Kastrinos F, Mukherjee B, Tayob N, et al. Risk of pancreatic cancer in families with Lynch syndrome. JAMA 2009;302:1790-5. [Crossref] [PubMed]
- Carrera S, Sancho A, Azkona E, et al. Hereditary pancreatic cancer: related syndromes and clinical perspective. Hered Cancer Clin Pract 2017;15:9. [Crossref] [PubMed]
- Giardiello FM, Offerhaus GJ, Lee DH, et al. Increased risk of thyroid and pancreatic carcinoma in familial adenomatous polyposis. Gut 1993;34:1394-6. [Crossref] [PubMed]
- Klein AP, Brune KA, Petersen GM, et al. Prospective risk of pancreatic cancer in familial pancreatic cancer kindreds. Cancer Res 2004;64:2634-8. [Crossref] [PubMed]
- Geoffroy-Perez B, Janin N, Ossian K, et al. Cancer risk in heterozygotes for ataxia-telangiectasia. Int J Cancer 2001;93:288-93. [Crossref] [PubMed]
- Thompson D, Easton DF. Cancer Incidence in BRCA1 mutation carriers. J Natl Cancer Inst 2002;94:1358-65. [Crossref] [PubMed]
- van Asperen CJ, Brohet RM, Meijers-Heijboer EJ, et al. Cancer risks in BRCA2 families: estimates for sites other than breast and ovary. J Med Genet 2005;42:711-9. [Crossref] [PubMed]
- Tenner S, Baillie J, DeWitt J, et al. American College of Gastroenterology guideline: management of acute pancreatitis. Am J Gastroenterol 2013;108:1400-15; 1416.
- Syngal S, Brand RE, Church JM, et al. ACG clinical guideline: Genetic testing and management of hereditary gastrointestinal cancer syndromes. Am J Gastroenterol 2015;110:223-62; quiz 263. [Crossref] [PubMed]
- Manero-Rupérez N, Martínez-Bosch N, Barranco LE, et al. The Galectin Family as Molecular Targets: Hopes for Defeating Pancreatic Cancer. Cells 2020;9:689. [Crossref] [PubMed]
- Martínez-Bosch N, Fernández-Barrena MG, Moreno M, et al. Galectin-1 drives pancreatic carcinogenesis through stroma remodeling and Hedgehog signaling activation. Cancer Res 2014;74:3512-24. [Crossref] [PubMed]
- Tang D, Zhang J, Yuan Z, et al. Pancreatic satellite cells derived galectin-1 increase the progression and less survival of pancreatic ductal adenocarcinoma. PLoS One 2014;9:e90476. [Crossref] [PubMed]
- Tang D, Yuan Z, Xue X, et al. High expression of Galectin-1 in pancreatic stellate cells plays a role in the development and maintenance of an immunosuppressive microenvironment in pancreatic cancer. Int J Cancer 2012;130:2337-48. [Crossref] [PubMed]
- Xue X, Lu Z, Tang D, et al. Galectin-1 secreted by activated stellate cells in pancreatic ductal adenocarcinoma stroma promotes proliferation and invasion of pancreatic cancer cells: an in vitro study on the microenvironment of pancreatic ductal adenocarcinoma. Pancreas 2011;40:832-9. [Crossref] [PubMed]
- Tang D, Gao J, Wang S, et al. Apoptosis and anergy of T cell induced by pancreatic stellate cells-derived galectin-1 in pancreatic cancer. Tumour Biol 2015;36:5617-26. [Crossref] [PubMed]
- Tang D, Zhang J, Yuan Z, et al. PSC-derived Galectin-1 inducing epithelial-mesenchymal transition of pancreatic ductal adenocarcinoma cells by activating the NF-κB pathway. Oncotarget 2017;8:86488-502. Erratum in: Oncotarget 2021;12:2111-3. [Crossref] [PubMed]
- Zhao W, Ajani JA, Sushovan G, et al. Galectin-3 Mediates Tumor Cell-Stroma Interactions by Activating Pancreatic Stellate Cells to Produce Cytokines via Integrin Signaling. Gastroenterology 2018;154:1524-1537.e6. [Crossref] [PubMed]
- Coppin L, Benomar K, Corfiotti F, et al. CA-125, but not galectin-3, complements CA 19-9 for discriminating ductal adenocarcinoma versus non-malignant pancreatic diseases. Pancreatology 2016;16:115-20. [Crossref] [PubMed]
- Jiang K, Lawson D, Cohen C, et al. Galectin-3 and PTEN expression in pancreatic ductal adenocarcinoma, pancreatic neuroendocrine neoplasms and gastrointestinal tumors on fine-needle aspiration cytology. Acta Cytol 2014;58:281-7. [Crossref] [PubMed]
- Daley D, Mani VR, Mohan N, et al. Dectin 1 activation on macrophages by galectin 9 promotes pancreatic carcinoma and peritumoral immune tolerance. Nat Med 2017;23:556-67. [Crossref] [PubMed]
- Yazdanifar M, Zhou R, Grover P, et al. Overcoming Immunological Resistance Enhances the Efficacy of A Novel Anti-tMUC1-CAR T Cell Treatment against Pancreatic Ductal Adenocarcinoma. Cells 2019;8:1070. [Crossref] [PubMed]
- Zhou W, Zhou Y, Chen X, et al. Pancreatic cancer-targeting exosomes for enhancing immunotherapy and reprogramming tumor microenvironment. Biomaterials 2021;268:120546. [Crossref] [PubMed]
- Seifert AM, Reiche C, Heiduk M, et al. Detection of pancreatic ductal adenocarcinoma with galectin-9 serum levels. Oncogene 2020;39:3102-13. [Crossref] [PubMed]
- Yee KO, Connolly CM, Duquette M, et al. The effect of thrombospondin-1 on breast cancer metastasis. Breast Cancer Res Treat 2009;114:85-96. [Crossref] [PubMed]
- Adams JC, Lawler J. The thrombospondins. Cold Spring Harb Perspect Biol 2011;3:a009712. [Crossref] [PubMed]
- Liao X, Wang W, Yu B, et al. Thrombospondin-2 acts as a bridge between tumor extracellular matrix and immune infiltration in pancreatic and stomach adenocarcinomas: an integrative pan-cancer analysis. Cancer Cell Int 2022;22:213. [Crossref] [PubMed]
- Kim J, Hoffman JP, Alpaugh RK, et al. An iPSC line from human pancreatic ductal adenocarcinoma undergoes early to invasive stages of pancreatic cancer progression. Cell Rep 2013;3:2088-99. [Crossref] [PubMed]
- Reinholt FP, Hultenby K, Oldberg A, et al. Osteopontin--a possible anchor of osteoclasts to bone. Proc Natl Acad Sci U S A 1990;87:4473-5. [Crossref] [PubMed]
- Lund SA, Giachelli CM, Scatena M. The role of osteopontin in inflammatory processes. J Cell Commun Signal 2009;3:311-22. [Crossref] [PubMed]
- Shang S, Plymoth A, Ge S, et al. Identification of osteopontin as a novel marker for early hepatocellular carcinoma. Hepatology 2012;55:483-90. [Crossref] [PubMed]
- Wang L, Zhou W, Zhong Y, et al. Overexpression of G protein-coupled receptor GPR87 promotes pancreatic cancer aggressiveness and activates NF-κB signaling pathway. Mol Cancer 2017;16:61. [Crossref] [PubMed]
- Zhao H, Chen Q, Alam A, et al. The role of osteopontin in the progression of solid organ tumour. Cell Death Dis 2018;9:356. [Crossref] [PubMed]
- Nallasamy P, Nimmakayala RK, Karmakar S, et al. Pancreatic Tumor Microenvironment Factor Promotes Cancer Stemness via SPP1-CD44 Axis. Gastroenterology 2021;161:1998-2013.e7. [Crossref] [PubMed]
- Kolb A, Kleeff J, Guweidhi A, et al. Osteopontin influences the invasiveness of pancreatic cancer cells and is increased in neoplastic and inflammatory conditions. Cancer Biol Ther 2005;4:740-6. [Crossref] [PubMed]
- Wang X, Abraham S, McKenzie JAG, et al. LRG1 promotes angiogenesis by modulating endothelial TGF-β signalling. Nature 2013;499:306-11. [Crossref] [PubMed]
- Cohen JD, Javed AA, Thoburn C, et al. Combined circulating tumor DNA and protein biomarker-based liquid biopsy for the earlier detection of pancreatic cancers. Proc Natl Acad Sci U S A 2017;114:10202-7. [Crossref] [PubMed]
- Lv S, Gao J, Zhu F, et al. Transthyretin, identified by proteomics, is overabundant in pancreatic juice from pancreatic carcinoma and originates from pancreatic islets. Diagn Cytopathol 2011;39:875-81. [Crossref] [PubMed]
- Aihara E, Engevik KA, Montrose MH. Trefoil Factor Peptides and Gastrointestinal Function. Annu Rev Physiol 2017;79:357-80. [Crossref] [PubMed]
- Perry JK, Kannan N, Grandison PM, et al. Are trefoil factors oncogenic? Trends Endocrinol Metab 2008;19:74-81. [Crossref] [PubMed]
- El Fitori J, Kleeff J, Giese NA, et al. Melanoma Inhibitory Activity (MIA) increases the invasiveness of pancreatic cancer cells. Cancer Cell Int 2005;5:3. [Crossref] [PubMed]
- Gebauer F, Wicklein D, Horst J, et al. Carcinoembryonic antigen-related cell adhesion molecules (CEACAM) 1, 5 and 6 as biomarkers in pancreatic cancer. PLoS One 2014;9:e113023. [Crossref] [PubMed]
- Simeone DM, Ji B, Banerjee M, et al. CEACAM1, a novel serum biomarker for pancreatic cancer. Pancreas 2007;34:436-43. [Crossref] [PubMed]
- Luo G, Jin K, Guo M, et al. Patients with normal-range CA19-9 levels represent a distinct subgroup of pancreatic cancer patients. Oncol Lett 2017;13:881-6. [Crossref] [PubMed]
- Topkan E, Selek U, Pehlivan B, et al. The Prognostic Significance of Novel Pancreas Cancer Prognostic Index in Unresectable Locally Advanced Pancreas Cancers Treated with Definitive Concurrent Chemoradiotherapy. J Inflamm Res 2021;14:4433-44. [Crossref] [PubMed]
- Awad S, Alkashash AM, Amin M, et al. Biochemical Predictors of Response to Neoadjuvant Therapy in Pancreatic Ductal Adenocarcinoma. Front Oncol 2020;10:620. [Crossref] [PubMed]
- Valukas C, Chawla A. Measuring response to neoadjuvant therapy using biomarkers in pancreatic cancer: a narrative review. Chin Clin Oncol 2022;11:30. [Crossref] [PubMed]
- Poruk KE, Gay DZ, Brown K, et al. The clinical utility of CA 19-9 in pancreatic adenocarcinoma: diagnostic and prognostic updates. Curr Mol Med 2013;13:340-51. [Crossref] [PubMed]
- Yin BW, Lloyd KO. Molecular cloning of the CA125 ovarian cancer antigen: identification as a new mucin, MUC16. J Biol Chem 2001;276:27371-5. [Crossref] [PubMed]
- Meng Q, Shi S, Liang C, et al. Diagnostic Accuracy of a CA125-Based Biomarker Panel in Patients with Pancreatic Cancer: A Systematic Review and Meta-Analysis. J Cancer 2017;8:3615-22. [Crossref] [PubMed]
- Groot VP, Rezaee N, Wu W, et al. Patterns, Timing, and Predictors of Recurrence Following Pancreatectomy for Pancreatic Ductal Adenocarcinoma. Ann Surg 2018;267:936-45. [Crossref] [PubMed]
- Parikh AA, Maiga A, Bentrem D, et al. Adjuvant Therapy in Pancreas Cancer: Does It Influence Patterns of Recurrence? J Am Coll Surg 2016;222:448-56. [Crossref] [PubMed]
- Daamen LA, Groot VP, Intven MPW, et al. Postoperative surveillance of pancreatic cancer patients. Eur J Surg Oncol 2019;45:1770-7. [Crossref] [PubMed]
- Motoi F, Murakami Y, Okada KI, et al. Sustained Elevation of Postoperative Serum Level of Carbohydrate Antigen 19-9 is High-Risk Stigmata for Primary Hepatic Recurrence in Patients with Curatively Resected Pancreatic Adenocarcinoma. World J Surg 2019;43:634-41. [Crossref] [PubMed]
- Massagué J, Obenauf AC. Metastatic colonization by circulating tumour cells. Nature 2016;529:298-306. [Crossref] [PubMed]
- Yeo D, Bastian A, Strauss H, et al. Exploring the Clinical Utility of Pancreatic Cancer Circulating Tumor Cells. Int J Mol Sci 2022;23:1671. [Crossref] [PubMed]
- Yadav DK, Bai X, Yadav RK, et al. Liquid biopsy in pancreatic cancer: the beginning of a new era. Oncotarget 2018;9:26900-33. [Crossref] [PubMed]
- Grunvald MW, Jacobson RA, Kuzel TM, et al. Current Status of Circulating Tumor DNA Liquid Biopsy in Pancreatic Cancer. Int J Mol Sci 2020;21:7651. [Crossref] [PubMed]
- Chen J, Wang H, Zhou L, et al. A combination of circulating tumor cells and CA199 improves the diagnosis of pancreatic cancer. J Clin Lab Anal 2022;36:e24341. [Crossref] [PubMed]
- Sefrioui D, Blanchard F, Toure E, et al. Diagnostic value of CA19.9, circulating tumour DNA and circulating tumour cells in patients with solid pancreatic tumours. Br J Cancer 2017;117:1017-25. [Crossref] [PubMed]