Specific metabolic impairments indicate loss of sustained liver improvements in metabolic dysfunction-associated steatotic liver disease treatment
Highlight box
Key findings
• Metabolic dysfunction-associated steatotic liver disease (MASLD) patients with insulin resistance (IR) and high total bile acid (TBA) levels are at higher risks of subsequent diminished sustained improvements of steatosis and fibrosis, respectively.
What is known and what is new?
• Progression and reversal of hepatic steatosis and fibrosis are critical to the prognosis of MASLD.
• This is the first cohort study to explore factors associated with loss of sustained liver improvement in MASLD treatment. IR at 24 weeks indicates subsequent diminished sustained hepatic steatosis improvements. High TBA level at 24 weeks might predict loss of sustained improvements in hepatic fibrosis. However, loss of hepatic steatosis response is not parallel to vanished hepatic fibrosis response.
What is the implication, and what should change now?
• MASLD patients who achieve an magnetic resonance imaging-based proton density fat fraction response but with IR should undergo intensive lifestyle interventions, as monitoring TBA levels and lowering it may benefit from delaying fibrosis progression.
Introduction
Metabolic dysfunction-associated steatotic liver disease (MASLD), renamed from nonalcoholic fatty liver disease (NAFLD), is the most prevalent etiology of chronic liver disease, impacting almost one-third of the general population worldwide (1,2). Unexpectedly, MASLD is rising in prevalence (increase of 29.1% up to 2030 compared to 2016), and the clinical burden of this unexpected increase is of great concern (3). Through its direct and indirect effect on disrupting glucose and lipid metabolism homeostasis by liver steatosis and subsequent liver injuries, MASLD not only induces deterioration from liver inflammation to fibrosis, liver decompensation and cancer, but also is a risk factor for the development of cardiovascular diseases, diabetes and stroke. As there remains no approval of a pharmacracy specific to MASLD, weight management to target is widely established as a first-line treatment (4-7).
For efficacy monitoring in MASLD, the gold standard of liver biopsy combined with histology scoring is limited by invasive procedures, related complications and sampling variations. Therefore, magnetic resonance imaging-based proton density fat fraction (MRI-PDFF), which quantifies the mobile proton density signal of triglycerides (TGs) and water noninvasively, has been accepted as an accurate alternative tool for repeated measurements of fat content within the liver. A meta-analysis of 1,100 patients derived from 13 cross-sectional studies demonstrated that its diagnostic value using liver biopsy as a reference can achieve an area under the summary receiver operating characteristic curve (AUC) of 0.91–0.98 for detecting steatosis grades of S1 to S3 (8). There is mounting evidence supporting that a ≥30% relative decline in MRI-PDFF is an imaging marker of disease activity resolution. One recent meta-analysis containing 346 subjects of clinical trials for MASLD found that MRI-PDFF response correlates positively with histologic remission response [pooled odds ratio (OR): 5.45; 95% confidence interval (CI): 1.53–19.46, P=0.009] during treatment (9). However, most studies in the aforementioned meta-analysis utilized a 24-week follow-up time point in the study design, and these studies did not incorporate information regarding the loss of sustained MRI-PDFF response when extended follow-up was performed.
Liver fibrosis estimation is another aspect of great clinical importance in MASLD management. Achieving fibrosis regression [fibrosis stages decrease 1 stage or more in the Meta-analysis of Histological Data in Viral Hepatitis (METAVIR) the steatosis, activity and fibrosis (SAF) scoring systems] or at least no worsening was proposed as a treatment endpoint in clinical trials. Shear wave elastography (SWE) is one of several noninvasive modalities for diagnosing liver fibrosis. A meta-analysis comprising 64 studies with 13,045 MASLD patients demonstrated that the pooled AUC of SWE could be 0.95 for advanced fibrosis, which is superior to other noninvasive indices or vibration-controlled transient elastography (VCTE) but comparable to magnetic resonance elastography (MRE) (10).
Understanding the association between the loss of MRI-PDFF response [no longer maintaining a 30% decline in liver fat content (LFC)] or the worsening of fibrosis compared to baseline and potential influencing factors in the subjects receiving therapy would help design more efficacious management strategies for MASLD, thus reducing the burden of disease progression. We conducted a large, prospective cohort study evaluating 48 weeks of weight loss treatment in patients with MASLD. Our primary aim was to assess the incidence of loss of response and identify potential predictors. We present this article in accordance with the STROBE reporting checklist (available at https://hbsn.amegroups.com/article/view/10.21037/hbsn-23-393/rc).
Methods
Study design and population
This was a prospective cohort study of MASLD patients diagnosed with MRI-PDFF at The First Affiliated Hospital of Sun Yat-sen University (Guangzhou, China). For this study, the baseline survey was conducted from January 2015 to February 2022 and followed up until April 2023. Of note, individuals with metabolic and alcohol-related/associated liver disease (MetALD) were not included in this study. The study was conducted in accordance with the Declaration of Helsinki (as revised in 2013). All participants provided signed informed consent prior to enrollment. This prospective study was approved by the Ethics Committee of The First Affiliated Hospital, Sun Yat-sen University {[2014]112}.
Subjects were included if they could be diagnosed with MASLD according to a multi-society Delphi consensus statement (1). The criteria include evidence of hepatic steatosis (hereby MRI-PDFF) in addition to at least one cardiometabolic risk factor. We excluded patients with any of the following exclusion criteria: (I) age <18 years; (II) other causes of liver disease, such as excessive alcohol intake (>210/140 g weekly in men/women), virus hepatitis, autoimmune liver disease and drug-induced liver injury; (III) decompensated cirrhosis; (IV) history or signs of malignancy, lung disease, heart disease or kidney disease; (V) glycated haemoglobin >9.0% or insulin usage; (VI) pregnancy or breastfeeding status; and (VII) incomplete information.
Clinical evaluation
A standard proforma questionnaire was conducted through face-to-face interviews to obtain the following information (supplementary file available at https://cdn.amegroups.cn/static/public/hbsn-23-393-1.pdf): demographic data, medical history, physical activity, alcohol intake and dietary habits. For the average daily diet composition, the daily diet information (such as meat, seafood, eggs, vegetables, fruit and nuts) was recorded and further calculated according to the Chinese Food Composition Tables (11,12). All participants also underwent physical examinations to determine their body height, weight, body mass index (BMI), waist circumference (WC), and blood pressure.
After fasting for a minimum of 8 hours, venous blood samples were collected to measure the following laboratory parameters using the Abbott c8000 Automatic Biochemistry Analyzer (Abbott, Abbott Park, IL, USA): liver biochemistry, lipid profiles, free fatty acid (FFA), uric acid (UA), fasting blood glucose (FBG) and insulin [fasting insulin (FINS)]. The levels of serum total bile acid (TBA) were measured by the enzyme circulation method (AU5800, Beckman Coulter, Brea, CA, USA). Hypertension was defined as high resting blood pressure levels ≥140/90 mmHg or the use of antihypertensive drugs (13). Hyperuricemia was defined as serum UA >420 μmol/L for males and >360 μmol/L for females. Homeostasis model assessment of insulin resistance (HOMA-IR) was calculated as [FINS (µU/mL) × FBG (mmol/L)]/22.5. Its cutoff value was 2.69 (14,15), which was utilized to identify insulin resistance (IR) in similar populations based on our previous study. Diabetes mellitus was defined as an FBG ≥7.0 mmol/L or a self-reported history (16).
Hepatic steatosis assessment
All participants underwent MRI-PDFF to diagnose fatty liver and evaluate average LFC using a 3.0-Tesla MRI scanner (Siemens 3.0 T Magnetom Verio; Siemens, Munchen, Germany). The fixed image analysts were blinded to all clinical data. The details of the MRI-PDFF protocol have been described previously and are briefly described as follows: time of echo 1 (TE1) 2.5 ms; TE2 3.7 ms; repetition time 5.47 ms; flip angle of 5°; ±504.0 kHz per pixel receiver bandwidth; and slice thickness, 3.0 mm (17). The cutoff values of hepatic fat accumulation were defined as LFC ≥5%, and its severity was classified as mild (<16.3%), moderate (16.3% to 21.7%) and severe (>21.7%) (18).
Liver stiffness measurements (LSM)
Two-dimensional SWE (2D-SWE) (Aix-en-Provence, France) was utilized to obtain liver stiffness measurements (LSM) by two fixed physicians who were blinded to the clinical information and had >5 years of experience with 2D-SWE measurements. The static SWE image was measured in a rectangular region of interest that was approximately 4 cm × 3 cm × 3 cm and set 1–2 cm below the surface of the liver, where a circular region of interest (the diameter set about 2.0 cm) without any focal lesion, biliary tracts, blood vessels, or artifacts from nearby lung gas or cardiac movement was selected. The means, minimum, maximum, and standard deviation (SD) of liver stiffness were obtained. The calculation of the average value of each participant was performed according to five consecutive 2D-SWE images, which were regarded as representative of the LSM (19). The cutoff values for discriminating different severities of liver fibrosis were defined as follows: F0 ≤6.3 kPa, F1 6.4–7.5 kPa, F2 7.6–8.8 kPa, F3 8.9–9.8 kPa, and F4 ≥9.9 kPa (20).
Follow-up and outcomes
During the follow-up, all subjects received surveillance and treatments from a multidisciplinary team, which included a hepatologist physician (B.Z.), a nutritional specialist (S.Z.), a cardiovascular physician (W.M.), an endocrinologist physician (X.C.) and a rheumatologist physician (L Liang). Comprehensive management flow of MASLD patients of lifestyle and pharmacracy with MRI-PDFF and 2D-SWE monitoring in this study was summarised in Figure 1. All patients were instructed by a nutritionist (S.Z.) to restrict carbohydrate and fat intake and to exercise with an easy-to-carry brochure recording personalized prescriptions (Figure S1A) according to the Dietary Reference Intakes and World Health Organization Global Strategy on Diet, Physical Activity and Health (21,22). Caloric restriction was defined as a reduction in daily energy (caloric) intake of 500–1,000 kcal/d from baseline (23). The nutritionist (S.Z.) estimated the patient’s daily caloric requirements based on their weight and level of physical activity, and then provided a dietary program that reduced patient’s daily energy intake by 500–1,000 kcal from the estimated caloric requirements (Figure S1B). The prescribed diets consisted of 60% carbohydrates, 15% protein, and 25% fat. Regular exercise was defined as participating in any kind of moderate-to-vigorous physical activity (MVPA) at least once a week (24,25). Total MVPA encompassed both recreational and occupational physical activity that lasted long enough to produce perspiration, such as bicycling, football, volleyball, carrying or lifting heavy loads, digging and construction work. On the structured questionnaires (supplementary file available at https://cdn.amegroups.cn/static/public/hbsn-23-393-1.pdf), participants provided information on the frequency, duration, and intensity of various physical activities. These activities were categorized into walking and MVPA based on the reported intensity (26). For individuals with indications for pharmacological therapy for lipid profiles, blood glucose or UAs, drug therapy was administered by supervising physicians based on the guidelines (13,27-29). Namely, this therapy comprised a statin for low-density lipoprotein cholesterol (LDL-C) control, a fenofibrate for TG control, angiotensin-converting enzyme inhibitors/angiotensin II receptor blockers (ACEI/ARB) or calcium channel blockers (CCB) for blood pressure control, glucagon-like peptide 1 receptor (GLP-1R), dipeptidyl peptidase 4 (DPP-4), sodium-dependent glucose transporters 2 (SGLT-2), metformin or pioglitazone for blood glucose control, and benzbromarone for UA control.
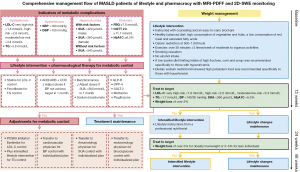
At weeks 0, 12, 24 and 48, all participants were scheduled for face-to-face visits at our center. During each visit, a structured lifestyle questionnaire, anthropometric parameters measurements and biochemical tests were conducted. If metabolic abnormalities remained uncontrolled or weight loss was less than 3% within 12 weeks, the multidisciplinary team would adjust medication prescriptions and provide intensified lifestyle intervention guidance. Intensified lifestyle interventions, implemented under the supervision of a clinical nutritionist (S.Z.), included individualized meal plans and longer durations of physical activity increased from 150 to 240 minutes of training per week (both aerobic and resistance training), the principles of which was detailed in Table S1.
At weeks 0, 24 and 48, MRI-PDFF and 2D-SWE were arranged to estimate the changes in LFC and LSM, respectively. MRI-PDFF response was defined as ≥30% relative decline of LFC values from baseline (8). For fibrosis regression evaluation, individuals with fibrosis stage 0 at baseline were removed from the analysis. LSM response was defined as ≥1 stage decline from baseline. The primary outcomes of the study were set as the loss of MRI-PDFF or LSM response at 48 weeks. Loss of MRI-PDFF response was determined as the absence of sustained MRI-PDFF response from week 24 to week 48, while loss of LSM response was defined as the absence of sustained LSM response from week 24 to week 48. Secondary outcomes included the changes from baseline in anthropological and biochemistry indicators, LFC quantified by MRI-PDFF and LSM determined by 2D-SWE at 24 and 48 weeks. The dynamic monitoring of these indicators was evaluated by absolute (Δ) and relative (Δ%) changes. For example, ΔLFCbaseline-24w = LFC at baseline − LFC at 24 weeks; ΔLFCbaseline-48w = LFC at baseline − LFC at 48 weeks; Δ%LFCbaseline-24w = (LFC at baseline − LFC at 24 weeks)/LFC at baseline ×100%. All endpoints were collected at both 24 and 48 weeks of follow-up.
Statistical analysis
All statistical analyses were performed using SPSS version 25.0 (IBM, Chicago, USA). Continuous variables are expressed as the mean ± SD or median [interquartile range (IQR)] and were compared using the independent samples t-test or the Wilcoxon-Mann-Whitney U test. Categorical variables are presented as frequencies (percentages) and were compared using the Chi-square test or Fisher’s exact test. The dynamic changes in outcome measures were investigated by repeated-measures analysis of variance. Notably, the normal upper limited value of TBA was set at a concentration of 4.1 μmol/L, which corresponded to the highest quartile concentration of TBA in our entire cohort. Univariable and multivariable logistic regression analyses were conducted to examine the risk factors for the loss of response in hepatic steatosis or fibrosis, and the multivariable adjustments included variables that were identified with statistic difference and potential confounders such as weight loss and IR status. Statistical significance was defined as two-sided P values <0.05.
Results
Patient characteristics
As shown in Figure 2, a total of 602 consecutive MASLD patients who underwent MRI-PDFF and 2D-SWE assessments were enrolled in this prospective analysis. The majority of the patients were male (73.3%), with a mean age of 41.1±13.6 years old. Of these patients, 201 received lipid-lowering drugs, 76 received uric-acid-lowering drugs, 47 received hypoglycemic drugs, 470 exercised regularly, 164 received intensified lifestyle intervention, and 319 achieved caloric restrictions (Table S2).
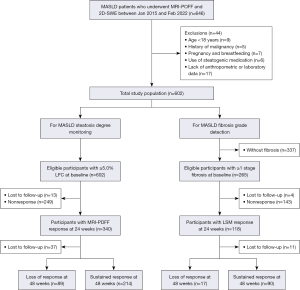
The dynamic changes in hepatic steatosis and fibrosis in every participant are outlined in Figure 3A,3B. After 24 weeks of follow-up, 57.7% (340/589) of MASLD patients presented MRI-PDFF response, 45.2% (118/261) of whom had LSM response concurrently. Regarding baseline clinical characteristics, subjects with MRI-PDFF response at 24 weeks exhibited significantly higher levels of FFA, HOMA-IR, alanine aminotransferase (ALT), aspartate aminotransferase (AST), γ-glutamyl transpeptidase (GGT), LFC and LSM but a higher proportion of regular exercise, caloric restriction than those with MRI-PDFF nonresponse (all P<0.05, Table S2). Compared with the patients without 24-week LSM response, those with 24-week LSM response tended to have higher baseline LDL-C, FINS, HOMA-IR and LFC levels but lower age, baseline FFA levels and the proportion of caloric restriction (all P<0.05, Table S2). Moreover, a lower prevalence of diabetes mellitus appeared in the LSM response group than in the LSM nonresponse (11.9% vs. 30.8%, P<0.001).
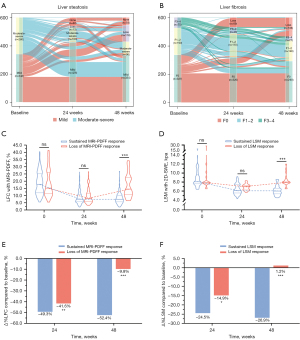
Clinical characteristic patterns of loss-response in steatosis and fibrosis
In patients with improved hepatic steatosis or fibrosis at 24 weeks, we followed up for an additional 24 weeks and divided them according to whether they maintained the response status of steatosis and fibrosis, respectively. Baseline characteristics of MASLD patients who stayed in the cohort and those who were lost to follow-up are presented in Table S3. For hepatic steatosis, 37 patients were lost to follow-up, and the rate of loss of MRI-PDFF response was 29.4% (89/303). Patients who experienced a loss of MRI-PDFF response and those who maintained a sustained MRI-PDFF response exhibited similar baseline in anthropometrical parameters, liver biochemistry, metabolic indicators, LFC, LSM, and lifestyle status at 0–24 weeks (Table 1). Regarding liver fibrosis, 11 subjects were lost to follow-up, and the rate of loss of LSM response was 15.9% (17/107). Compared with the sustained LSM response group, higher baseline WC and FBG levels were observed in the loss of LSM response group (all P<0.05, Table 1).
Table 1
Characteristics | Liver steatosis | Liver fibrosis | |||||||
---|---|---|---|---|---|---|---|---|---|
Total† (n=303) |
Sustained MRI-PDFF response at 48 weeks (n=214) | Loss of MRI-PDFF response at 48 weeks (n=89) | P | Total‡ (n=107) |
Sustained LSM response at 48 weeks (n=90) |
Loss of LSM response at 48 weeks (n=17) |
P | ||
Age (years) | 42.0±13.9 | 42.9±13.9 | 39.8±13.9 | 0.08 | 42.1±11.3 | 42.4±11.6 | 40.7±10.1 | 0.56 | |
Male | 221 (72.9) | 157 (73.4) | 64 (71.9) | 0.80 | 77 (72.0) | 62 (68.9) | 15 (88.2) | 0.18 | |
Weight (kg) | 76.7±11.6 | 76.4±11.3 | 77.5±12.4 | 0.43 | 77.1±11.2 | 76.5±11.4 | 80.7±9.5 | 0.15 | |
BMI (kg/m2) | 27.7±3.3 | 27.6±3.2 | 27.8±3.6 | 0.61 | 28.2±3.2 | 27.9±3.1 | 29.42± 3.5 | 0.08 | |
WC (cm) | 91.7±8.0 | 91.6±7.9 | 92.0±8.3 | 0.69 | 92.8±7.6 | 92.1±7.2 | 96.2±8.7 | 0.04 | |
Waist-hip ratio | 0.90±0.05 | 0.90±0.05 | 0.91±0.05 | 0.68 | 0.91±0.05 | 0.91±0.05 | 0.93±0.06 | 0.15 | |
CHOL (mmol/L) | 5.08±1.08 | 5.09±1.05 | 5.06±1.16 | 0.84 | 5.04±0.91 | 5.08±0.93 | 4.84±0.78 | 0.33 | |
TG (mmol/L) | 1.68 (1.18, 2.27) | 1.74 (1.29, 2.36) | 1.50 (1.14, 2.12) | 0.06 | 1.69 (1.32, 2.20) | 1.71 (1.34, 2.23) | 1.30 (1.01, 2.00) | 0.15 | |
HDL-C (mmol/L) | 1.14±0.27 | 1.14±0.25 | 1.14±0.32 | 0.78 | 1.10±0.23 | 1.10±0.23 | 1.08±0.21 | 0.66 | |
LDL-C (mmol/L) | 3.20±0.80 | 3.22±0.77 | 3.17±0.86 | 0.62 | 3.25±0.70 | 3.28±0.71 | 3.12±0.63 | 0.39 | |
FFA (mmol/L) | 541 (438, 739) | 521 (437, 739) | 551 (446, 738) | 0.42 | 508 (424, 691) | 497 (413, 660) | 599 (476, 827) | 0.06 | |
FBG (mmol/L) | 5.0 (4.6, 5.7) | 5.0 (4.6, 5.7) | 4.9 (4.6, 5.9) | 0.89 | 5.0 (4.6, 6.0) | 5.0 (4.6, 5.7) | 6.0 (4.9, 6.7) | 0.02 | |
FINS (μU/mL) | 11.0 (8.5, 14.9) | 11.0 (8.5, 14.6) | 11.7 (8.5, 15.6) | 0.41 | 12.8 (9.7, 19.9) | 12.8 (9.6, 20.8) | 10.1 (9.2, 14.7) | 0.14 | |
HOMA-IR | 2.65 (1.85, 3.70) | 2.65 (1.91, 3.62) | 2.69 (1.79, 3.93) | 0.50 | 2.80 (2.20, 4.40) | 2.89 (2.17, 4.89) | 2.75 (2.09, 4.01) | 0.78 | |
UA (μmol/L) | 433.2±100.9 | 428.7±97.9 | 444.2±107.6 | 0.22 | 404.4±95.1 | 401.5±101.2 | 419.8±51.3 | 0.27 | |
ALT (U/L) | 50.0 (32.0, 83.0) | 50.0 (32.0, 81.5) | 54.0 (31.0, 88.5) | 0.78 | 38.0 (30.0, 72.0) | 38.0 (30.0, 72.0) | 38.0 (29.0, 96.0) | 0.88 | |
AST (U/L) | 36.0 (26.0, 56.0) | 36.0 (26.8, 55.0) | 35.0 (24.5, 59.0) | 0.51 | 34.0 (22.0, 51.0) | 36.5 (22.0, 50.3) | 27.0 (22.0, 57.0) | 0.43 | |
GGT (U/L) | 47.0 (30.0, 72.0) | 50.0 (29.0, 72.0) | 44.0 (32.0, 59.8) | 0.52 | 40.5 (29.8, 61.0) | 40.0 (29.0, 59.5) | 43.0 (29.5, 76.5) | 0.76 | |
ALP (U/L) | 78.0 (67.0, 91.0) | 78.0 (64.8, 93.0) | 78.0 (68.0, 87.8) | 0.86 | 78.0 (67.0, 90.0) | 78.0 (67.0, 94.3) | 74.0 (67.0, 81.0) | 0.26 | |
Albumin (g/L) | 45.9±3.2 | 45.9±3.1 | 46.0±3.3 | 0.85 | 45.6±2.9 | 45.6±3.1 | 45.7±1.9 | 0.83 | |
TB (μmol/L) | 12.7 (10.2, 16.2) | 12.6 (10.2, 16.1) | 13.0 (10.1, 16.8) | 0.35 | 12.3 (10.0, 17.0) | 12.2 (10.0, 16.5) | 16.3 (10.0, 24.2) | 0.09 | |
TBA (μmol/L) | 2.6 (1.8, 4.1) | 2.6 (1.8, 3.9) | 2.6 (1.8, 4.8) | 0.89 | 2.7 (2.0, 4.1) | 2.7 (2.0, 4.0) | 2.6 (1.6, 5.5) | 0.89 | |
LFC (%) | 17.5 (11.7, 25.5) | 17.8 (11.9, 25.1) | 15.2 (11.2, 26.2) | 0.51 | 18.3 (11.3, 24.3) | 18.9 (11.1, 24.3) | 14.7 (11.7, 27.5) | 0.94 | |
LSM (kpa) | 6.7 (5.6, 8.0) | 6.7 (5.6, 8.0) | 6.7 (5.5, 8.4) | 0.69 | 8.0 (7.6, 9.6) | 8.1 (7.6, 9.6) | 7.9 (7.7, 8.6) | 0.63 | |
Hypertension | 84 (27.7) | 54 (25.2) | 30 (33.7) | 0.12 | 28 (26.2) | 23 (25.6) | 5 (29.4) | 0.98 | |
Diabetes mellitus | 57 (18.8) | 37 (17.3) | 20 (22.5) | 0.30 | 14 (13.1) | 12 (13.3) | 2 (11.8) | 1.00 | |
Smoking | 26 (8.6) | 21 (9.8) | 5 (5.6) | 0.27 | 4 (3.7) | 3 (3.3) | 1 (5.9) | 0.51 | |
Regular exercise§ | 265 (87.5) | 190 (88.8) | 75 (84.3) | 0.36 | 87 (81.3) | 74 (82.2) | 13 (76.5) | 1.00 | |
Caloric restriction§ | 196 (64.7) | 142 (66.4) | 54 (60.7) | 0.40 | 53 (49.5) | 48 (53.3) | 5 (29.4) | 0.09 | |
Lipid-lowering drug | 102 (33.7) | 69 (32.2) | 33 (37.1) | 0.43 | 40 (37.4) | 34 (37.8) | 6 (35.3) | 0.82 | |
Hypoglycemic drug | 30 (9.9) | 19 (8.9) | 11 (12.4) | 0.33 | 6 (5.6) | 6 (6.7) | 0 (0.0) | 0.59 | |
Uric-acid-lowering drug | 45 (14.9) | 26 (12.1) | 19 (21.3) | 0.06 | 14 (13.1) | 10 (11.1) | 4 (23.5) | 0.33 | |
Intensified lifestyle intervention | 86 (28.4) | 60 (28.0) | 26 (29.2) | 0.87 | 33 (30.8) | 31 (34.4) | 2 (11.8) | 0.06 |
Values are expressed as mean ± standard deviation, median (interquartile range) or n (%). †, 303 MASLD patients who showed MRI-PDFF response at 24 weeks and continued to complete a further 24-week follow-up; ‡, 107 MASLD patients who showed LSM response at 24 weeks and continued to complete a further 24-week follow-up; §, the lifestyle status at 0–24 weeks after enrollment. Caloric restriction was defined as a reduction in energy (caloric) intake of 500–1,000 kcal/day from baseline; regular exercise was defined as moderate-to-vigorous physical activity at least once a week. MASLD, metabolic dysfunction-associated steatotic liver disease; MRI-PDFF, magnetic resonance imaging-based proton density fat fraction; LSM, liver stiffness measurement; BMI, body mass index; WC, waist circumference; CHOL, total cholesterol; TG, triglyceride; HDL-C, high-density lipoprotein cholesterol; LDL-C, low-density lipoprotein cholesterol; FFA, free fatty acid; FBG, fasting blood glucose; FINS, fasting insulin; HOMA-IR, homeostasis model assessment of insulin resistance; UA, uric acid; ALT, alanine aminotransferase; AST, aspartate aminotransferase; GGT, γ-glutamyl transpeptidase; ALP, alkaline phosphatase; TB, total bilirubin; TBA, total bile acid; LFC, liver fat content.
The absolute changes in clinical indicators are detailed in Tables S4,S5. Among the loss of MRI-PDFF response group, 24-week changes in weight, BMI, WC, FFA, FBG, UA, ALT, AST, GGT, and LFC were higher than 48-week changes (all P<0.05, Table S4). Among the loss of LSM response group, changes in LSM were higher at 24 weeks than at 48 weeks [1.00 (IQR, 0.55, 2.30) vs. −0.10 (IQR, −0.35, 0.08) kpa, P<0.001, Table S5]. Notably, when comparing 24-week changes between patients without and with sustained MRI-PDFF response at 48 weeks, significant differences were not observed (all P>0.05, Table S4). However, individuals with loss of LSM response tended to have a larger decrease in total bilirubin but not in alkaline phosphatase, TBA and LSM (all P<0.05, Table S5).
Furthermore, we analyzed the dynamic changes in LFC and LSM (Figure 3). The median LFC values in patients with loss of MRI-PDFF response status were 15.2%, 8.0% and 14.5% at 0, 24 and 48 weeks, respectively. On the other hand, patients with sustained MRI-PDFF response status contained median LFC values of 17.8%, 7.5% and 7.4% at each corresponding follow-up point, respectively. At 24 weeks, the median LFC levels between the two groups did not reach a significant difference (Figure 3C), while the relative decline ratio of LFC was lower in the loss of MRI-PDFF response group than in the sustained MRI-PDFF response group at 24 weeks (−41.6% vs. −49.3%, P=0.001, Figure 3E). At 48 weeks, both the median LFC and relative decline ratio between the two groups showed significant differences (all P<0.001, Figure 3C,3E). A similar trend was also shown in the median LSM values and corresponding relative decline ratios (Figure 3D,3F).
Predictors of loss of response in hepatic steatosis
The univariate analysis in patients with MRI-PDFF response at 24 weeks revealed that 24-week IR, still regular exercise and caloric restriction after 24 weeks, Δ%LDL-Cbaseline-24w 10% category and Δ%LFCbaseline-24w 10% category were associated with the loss of response of hepatic steatosis. After multivariable adjustments, 24-week IR (OR: 2.97, 95% CI: 1.37–6.41, P=0.006), regular exercise (OR: 0.36, 95% CI: 0.16–0.80, P=0.01) and caloric restriction (OR: 0.29, 95% CI: 0.15–0.55, P<0.001) after 24 weeks, and Δ%LFCbaseline-24w 10% category (OR: 0.75, 95% CI: 0.58–0.96, P=0.02) remained significantly and independently associated with the loss of response in hepatic steatosis (Table 2). As shown in Figure 4, different longitudinal IR patterns exhibited different dynamic changes in LFC measured by MRI-PDFF. Individuals with IR at baseline had more severe hepatic steatosis, while those with IR at 24 weeks tended to have a subsequent diminished sustained response in hepatic steatosis (Figure 4A).
Table 2
Predictors | Loss of steatosis response with MRI-PDFF (n=303) | Loss of fibrosis response with 2D-SWE (n=107) | |||||||||
---|---|---|---|---|---|---|---|---|---|---|---|
Univariate | Multivariate | Univariate | Multivariate | ||||||||
OR (95% CI) | P | OR (95% CI) | P | OR (95% CI) | P | OR (95% CI) | P | ||||
Age 10-year category | 0.85 (0.71–1.02) | 0.08 | – | – | 0.87 (0.55–1.38) | 0.56 | – | – | |||
Sex (male) | 0.93 (0.54–1.62) | 0.80 | – | – | 3.39 (0.73–15.82) | 0.12 | – | – | |||
Hypertension† (yes) | 1.53 (0.90–2.63) | 0.12 | – | – | 1.21 (0.39–3.82) | 0.74 | – | – | |||
Diabetes mellitus (yes) | 1.38 (0.75–2.54) | 0.30 | – | – | 0.86 (0.17–4.22) | 0.85 | – | – | |||
Smoking (yes) | 0.57 (0.21–1.57) | 0.28 | – | – | 1.81 (0.18–18.54) | 0.62 | – | – | |||
Indicators at baseline | |||||||||||
Weight (kg) | 1.01 (0.99–1.03) | 0.43 | – | – | 1.03 (0.99–1.08) | 0.15 | – | – | |||
BMI (kg/m2) | 1.02 (0.95–1.10) | 0.61 | – | – | 1.16 (0.98–1.37) | 0.08 | – | – | |||
WC (cm) | 1.01 (0.98–1.04) | 0.69 | – | – | 1.08 (1.002–1.17) | 0.04 | 0.94 (0.83–1.06) | 0.32 | |||
TG (mmol/L) | 0.89 (0.70–1.14) | 0.35 | – | – | 0.94 (0.52–1.73) | 0.85 | – | – | |||
HDL-C (mmol/L) | 0.88 (0.35–2.21) | 0.78 | – | – | 0.59 (0.06–6.14) | 0.66 | – | – | |||
LDL-C (mmol/L) | 0.92 (0.68–1.26) | 0.62 | – | – | 0.70 (0.32–1.55) | 0.38 | – | – | |||
FFA 100 mmol/L category | 1.06 (0.93–1.20) | 0.41 | – | – | 1.36 (1.01–1.82) | 0.04 | 1.06 (0.65–1.75) | 0.81 | |||
FBG (mmol/L) | 1.19 (0.97–1.46) | 0.09 | – | – | 1.38 (0.93–2.07) | 0.11 | – | – | |||
FINS (μU/mL) | 1.00 (0.97–1.04) | 0.78 | – | – | 0.93 (0.85–1.02) | 0.12 | – | – | |||
Insulin resistance† | 1.34 (0.81–2.20) | 0.25 | 1.04 (0.50–2.17) | 0.91 | 1.68 (0.54–5.16) | 0.37 | 3.66 (0.50–26.58) | 0.20 | |||
Hyperuricemia† | 1.67 (0.98–2.82) | 0.06 | – | – | 1.41 (0.50–3.98) | 0.52 | – | – | |||
TBA (μmol/L) | 0.97 (0.88–1.07) | 0.49 | – | – | 1.00 (0.89–1.13) | 0.94 | – | – | |||
ALT 10 IU/L category | 1.00 (0.95–1.06) | 0.91 | – | – | 0.98 (0.85–1.13) | 0.77 | – | – | |||
GGT 10 IU/L category | 0.98 (0.94–1.02) | 0.34 | – | – | 0.99 (0.89–1.10) | 0.87 | – | – | |||
ALP 10 IU/L category | 0.95 (0.88–1.01) | 0.12 | – | – | 0.79 (0.58–1.08) | 0.14 | – | – | |||
LFC 5.0% category | 1.00 (0.86–1.16) | 0.98 | – | – | 0.99 (0.73–1.35) | 0.96 | – | – | |||
LSM (kpa) | 0.95 (0.86–1.04) | 0.27 | – | – | 1.03 (0.89–1.21) | 0.68 | – | – | |||
Regular exercise at 0–24 weeks | 0.69 (0.31–1.54) | 0.37 | – | – | 0.78 (0.19–3.16) | 0.73 | – | – | |||
Caloric restriction at 0–24 weeks | 0.78 (0.44–1.39) | 0.40 | – | – | 0.35 (0.10–1.22) | 0.10 | – | – | |||
Indicators at 24 weeks | – | – | – | – | |||||||
Weight (kg) | 1.01 (0.97–1.03) | 0.47 | – | – | 1.03 (0.98–1.08) | 0.28 | – | – | |||
BMI (kg/m2) | 1.02 (0.94–1.10) | 0.70 | – | – | 1.14 (0.94–1.37) | 0.18 | – | – | |||
WC (cm) | 0.99 (0.96–1.03) | 0.61 | – | – | 1.05 (0.97–1.14) | 0.20 | – | – | |||
TG (mmol/L) | 0.82 (0.57–1.19) | 0.29 | – | – | 0.79 (0.31–2.03) | 0.63 | – | – | |||
HDL-C (mmol/L) | 0.99 (0.43–2.28) | 0.98 | – | – | 0.98 (0.10–9.77) | 0.99 | – | – | |||
LDL-C (mmol/L) | 1.45 (0.95–2.20) | 0.08 | – | – | 1.20 (0.53–2.73) | 0.67 | – | – | |||
FFA 100 mmol/L category | 0.93 (0.81–1.07) | 0.31 | – | – | 1.29 (0.98–1.69) | 0.07 | – | – | |||
FBG (mmol/L) | 1.10 (0.82–1.47) | 0.54 | – | – | 1.31 (0.76–2.25) | 0.33 | – | – | |||
FINS (μU/mL) | 1.01 (0.98–1.04) | 0.46 | – | – | 0.89 (0.78–1.03) | 0.12 | – | – | |||
Insulin resistance† | 2.37 (1.40–4.00) | 0.001 | 2.97 (1.37–6.41) | 0.006 | 0.43 (0.11–1.61) | 0.21 | 0.38 (0.06–2.51) | 0.32 | |||
Hyperuricemia† | 1.03 (0.63–1.69) | 0.91 | – | – | 0.92 (0.32–2.62) | 0.87 | – | – | |||
TBA (μmol/L) | 0.98 (0.93–1.04) | 0.56 | – | – | 1.60 (1.25–2.06) | <0.001 | 1.41 (1.04–1.92) | 0.03 | |||
ALT 10 IU/L category | 0.88 (0.75–1.04) | 0.12 | – | – | 0.81 (0.54–1.23) | 0.33 | – | – | |||
GGT 10 IU/L category | 0.97 (0.92–1.03) | 0.33 | – | – | 1.04 (0.91–1.20) | 0.54 | – | – | |||
ALP 10 IU/L category | 0.93 (0.85–1.01) | 0.10 | – | – | 0.94 (0.68–1.29) | 0.69 | – | – | |||
LFC 5.0% category | 1.25 (0.96–1.63) | 0.10 | – | – | 0.92 (0.57–1.49) | 0.73 | – | – | |||
LSM (kpa) | 0.92 (0.79–1.08) | 0.30 | – | – | 1.44 (0.91–2.29) | 0.12 | – | – | |||
Regular exercise after 24 weeks | 0.32 (0.16–0.65) | 0.002 | 0.36 (0.16–0.80) | 0.01 | 0.65 (0.12–3.52) | 0.62 | – | – | |||
Caloric restriction after 24 weeks | 0.33 (0.19–0.60) | <0.001 | 0.29 (0.15–0.55) | <0.001 | 0.24 (0.05–1.19) | 0.08 | – | – | |||
Changes of indicators‡ | |||||||||||
Δ%Weightbaseline-24w 10% category | 1.06 (0.61–1.83) | 0.84 | 1.98 (0.89–4.41) | 0.10 | 1.36 (0.53–3.49) | 0.53 | 0.35 (0.08–1.55) | 0.17 | |||
Δ%WCbaseline-24w 10% category | 1.48 (0.87–2.53) | 0.15 | – | – | 1.91 (0.63–5.77) | 0.25 | – | – | |||
Δ%TGbaseline-24w 10% category | 0.99 (0.93–1.05) | 0.69 | – | – | 0.92 (0.79–1.06) | 0.23 | – | – | |||
Δ%HDL-Cbaseline-24w 10% category | 0.93 (0.81–1.06) | 0.25 | – | – | 0.92 (0.65–1.31) | 0.65 | – | – | |||
Δ%LDL-Cbaseline-24w 10% category | 0.91 (0.83–0.99) | 0.04 | 0.90 (0.81–1.002) | 0.053 | 0.87 (0.68–1.11) | 0.26 | – | – | |||
Δ%FFAbaseline-24w 10% category | 1.06 (0.98–1.13) | 0.15 | – | – | 0.99 (0.90–1.09) | 0.83 | – | – | |||
Δ%FBGbaseline-24w 10% category | 1.13 (0.93–1.37) | 0.21 | – | – | 1.33 (0.92–1.92) | 0.13 | – | – | |||
Δ%FINSbaseline-24w 10% category | 0.97 (0.91–1.05) | 0.46 | – | – | 1.04 (0.88–1.23) | 0.63 | – | – | |||
Δ%HOMA-IRbaseline-24w 10% category | 0.99 (0.93–1.06) | 0.77 | – | – | 1.08 (0.93–1.26) | 0.33 | – | – | |||
Δ%UAbaseline-24w 10% category | 0.97 (0.87–1.09) | 0.61 | – | – | 1.06 (0.83–1.35) | 0.66 | – | – | |||
Δ%TBAbaseline-24w 10% category | 0.99 (0.96–1.01) | 0.31 | – | – | 0.87 (0.81–0.93) | <0.001 | 0.84 (0.75–0.95) | 0.006 | |||
Δ% ALTbaseline-24w 10% category | 1.03 (0.97–1.10) | 0.33 | – | – | 0.98 (0.88–1.09) | 0.70 | – | – | |||
Δ%GGTbaseline-24w 10% category | 0.98 (0.91–1.06) | 0.68 | – | – | 0.93 (0.81–1.08) | 0.35 | – | – | |||
Δ%ALPbaseline-24w 10% category | 1.06 (0.93–1.19) | 0.40 | – | – | 0.72 (0.50–1.03) | 0.07 | – | – | |||
Δ%LFCbaseline-24w 10% category | 0.76 (0.64–0.92) | 0.004 | 0.75 (0.58–0.96) | 0.02 | 0.90 (0.78–1.04) | 0.15 | – | – | |||
Δ%LSMbaseline-24w 10% category | 1.07 (0.91–1.25) | 0.41 | – | – | 0.69 (0.46–1.04) | 0.08 | – | – | |||
Loss of MRI-PDFF response | – | – | – | – | 1.20 (0.28–5.05) | 0.81 | – | – |
†, hypertension was defined as average blood pressure levels ≥140/90 mmHg or use of hypertensive medication; insulin resistance was HOMA-IR >2.69. Hyperuricemia was defined as serum uric acid >420 μmol/L for male and >360 μmol/L for female; caloric restriction was defined as a reduction in energy (caloric) intake of 500–1,000 kcal/day from baseline; regular exercise was defined as moderate-to-vigorous physical activity at least once a week; ‡, Δ%BMIbaseline-24w 10% category was not included in this table because the relative changes in weight and BMI are identical. MASLD, metabolic dysfunction-associated steatotic liver disease; MRI-PDFF, magnetic resonance imaging-based proton density fat fraction; 2D-SWE, two-dimensional shear wave elastography; OR, odds ratio; 95% CI, 95% confidence interval; BMI, body mass index; WC, waist circumference; TG, triglyceride; HDL-C, high-density lipoprotein cholesterol; LDL-C, low-density lipoprotein cholesterol; FFA, free fatty acid; FBG, fasting glucose; FINS, fasting insulin; TBA, total bile acid; ALT, alanine aminotransferase; GGT, γ-glutamyl transpeptidase; ALP, alkaline phosphatase; LFC, liver fat content; LSM, liver stiffness measurement; HOMA-IR, homeostasis model assessment of insulin resistance; UA, uric acid; Δ%, relative change from baseline.
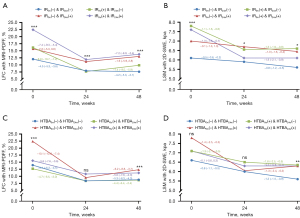
To further explore the association of weight change, IR status, and gender with the sustained efficacy of hepatic steatosis, the participants who exhibited MRI-PDFF response at 24 weeks were subgrouped and analyzed by multivariable analysis. Subgroup clinical characteristics are provided in Tables S6-S8. Multivariable logistic regression model confirmed that 24-week IR (OR: 3.33, 95% CI: 1.40–7.90, P=0.006), regular exercise after 24 weeks (OR: 0.22, 95% CI: 0.08–0.62, P=0.004) and Δ%LFCbaseline-24w 10% category (OR: 0.63, 95% CI: 0.43–0.94, P=0.02) were risk factors for the loss of MRI-PDFF response at 48 weeks in the subgroup whose weight loss was <5% from baseline to 24 weeks (Figure 5A), while only caloric restriction after 24 weeks (OR: 0.19, 95% CI: 0.07–0.54, P=0.002) was a risk factor in those with weight loss of ≥5% (Figure 5B). A sub-analysis of the subjects without IR at baseline showed that 24-week IR (OR: 8.17, 95% CI: 1.50–44.60, P=0.02) and regular exercise after 24 weeks (OR: 0.14, 95% CI: 0.04–0.43, P=0.001) remained significant factors (Figure 5C). Among the subjects with IR at baseline, multivariable analysis showed that 24-week IR (OR: 2.91, 95% CI: 1.17–7.22, P=0.02) and caloric restriction after 24 weeks (OR: 0.33, 95% CI: 0.13–0.87, P=0.02) were independent predictors of hepatic steatosis fluctuations (Figure 5D). In male patients, significant predictors of the loss of MRI-PDFF response included hypertension (OR: 3.25, 95% CI: 1.30–8.17, P=0.01), ALT 10-IU/L category (OR: 0.75, 95% CI: 0.57–0.99, P=0.04), and still regular exercise (OR: 0.23, 95% CI: 0.09–0.58, P=0.002) and caloric restriction after 24 weeks (OR: 0.43, 95% CI: 0.20–0.93, P=0.03) (Figure S2A). For female patients, significant predictors were caloric restriction after 24 weeks (OR: 0.07, 95% CI: 0.01–0.65, P=0.02) and Δ%LFCbaseline-24w 10% category (OR: 0.39, 95% CI: 0.20–0.80, P=0.01) (Figure S2B).
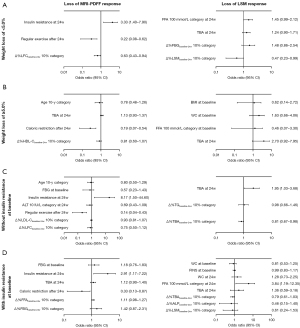
Predictors of loss of response in hepatic fibrosis
Univariate analysis of patients with 24-week LSM response showed that the baseline WC and FFA, 24-week TBA and Δ%TBAbaseline-24w were significantly associated with the loss of LSM response. Further multivariable analysis demonstrated that 24-week serum TBA levels (OR: 1.41, 95% CI: 1.04–1.92, P=0.03) and Δ%TBAbaseline-24w 10% category (OR: 0.84, 95% CI: 0.75–0.95, P=0.006) were the independent risk factors for the loss of response in hepatic fibrosis (Table 2). As presented in Figure 4D, different longitudinal TBA patterns affected the evolution of LSM, and subjects with high TBA levels at 24 weeks (≥4.1 μmol/L) tended to lose response to liver fibrosis.
Furthermore, subgroup analyses were conducted and baseline characteristics are detailed in Tables S6-S8. After subgrouping by weight loss ≥5% at 0–24 weeks, multivariable analysis only found that Δ%LSMbaseline-24w 10% category (OR: 0.47, 95% CI: 0.23–0.99, P=0.04) was significant in predicting the loss of LSM response among the individuals with weight loss of <5% (Figure 5A). After stratifying by baseline IR status, multivariable logistic regression model showed that 24-week TBA (OR: 1.95, 95% CI: 1.03–3.68, P=0.04) and Δ%TBAbaseline-24w 10% category (OR: 0.81, 95% CI: 0.67–0.98, P=0.03) were risk factors for the loss of LSM response in the individuals without IR at baseline (Figure 5C), while only 24-week FFA 100-mmol/L category (OR: 3.84, 95% CI: 1.19–12.35, P=0.02) was a risk factor in those with IR at baseline (Figure 5D). A subgroup analysis of male patients showed that FBG at baseline (OR: 3.45, 95% CI: 1.20–9.91, P=0.02) and Δ%TBAbaseline-24w 10% category (OR: 0.86, 95% CI: 0.76–0.97, P<0.001) were significant predictors of hepatic fibrosis fluctuations (Figure S2A). However, no statistically significant variables were identified in the univariate logistic regression analysis for female patients, and therefore multivariable analysis was not conducted.
Association between MRI-PDFF response and LSM response
The relationship of MRI-PDFF response with LSM response was examined. At 24 weeks, individuals with MRI-PDFF response showed a higher proportion of LSM response compared to those with MRI-PDFF nonresponse (50.0% vs. 35.6%, P=0.03, Figure S3A). Additionally, a relative decline in LFC of ≥30% at 24 weeks was significantly associated with a decline in LSM of ≥1 stage in a multivariable logistic regression model controlling for age and gender, with an OR of 1.92 (95% CI: 1.11–3.31, P=0.02, Figure S3B). However, at 48 weeks, there was no difference in the rate of loss of LSM response between MASLD patients with and without loss of MRI-PDFF response (15.8% vs. 13.6%, P=0.81, Figure S3C). Furthermore, loss of MRI-PDFF response at 48 weeks was not found be a risk factor for the loss of LSM response (OR: 1.20, 95% CI: 0.28–5.05, P=0.81, Table 2, Figure S3D).
Discussion
Utilizing a large prospective longitudinal cohort of 602 MASLD patients receiving treatment with paired MRI-PDFF and 2D-SWE estimations, we observed that after another 24 weeks from the 24-week time point of baseline, the incidence of secondary loss of response to hepatic steatosis and fibrosis was 29.4% and 15.9%, respectively. Additionally, persistent IR, lack of regular exercise and caloric restriction after 24 weeks, and lower Δ%LFCbaseline-24w were noted for the incidences of loss of response in hepatic steatosis, while higher TBA levels and lower Δ%TBAbaseline-24w were associated with increasing failure of sustained response in hepatic fibrosis. Thus, our findings indicated that MASLD patients would experience sustained benefits to their liver improvements by lessening IR, lowering TBA levels and modifying lifestyle.
This is the first study to utilize the loss of sustained MRI-PDFF response as an end-point in MASLD. According to a review summarizing 52 weeks of line in MASLD, only approximately 9–12% of patients achieved weight loss over 5% to target and experienced histologic benefit (30). As a chronic disease, relying on long-term weight loss management has become the major obstacle in its therapy, mainly because there remains no specific pharmacracy available. A few impactors of loss of response in steatosis were identified in our observations. Similar to correctional study demonstrating that IR was independently associated with steatosis grades (31), our findings suggested that residual IR might induce subsequent steatosis progression, especially in MASLD patients with unsuccessful weight loss. However, this conclusion should be interpreted with caution since the IR measurements did not adopt the gold standard of the glucose clamp technique in this study. Notably, IR is characterized by insensately of target organs such as muscle, liver or adiposity to insulin and compensated high insulinemia, the latter of which could both directly activate de novo fatty acid generation and indirectly fat mass lipolysis, inducing overaccumulation of intrahepatic fat (31).
Altered enterohepatic circulation of bile acid has been recognized as a marker of liver damage. TBA levels and compositions can be affected by either one of the changes in the synthetic, transported, secretory or reabsorption abilities of the liver (32). Serum bile acids are derived from TBA pool that leak from the bile or intestinal tract into the circulation. High concentrations of TBA can bind to several receptors among hepatocytes, macrophages, ileal enterocytes, and hepatic stellate cells, such as farnesoid X receptor, protein-coupled receptor 5, vitamin D receptor and sphingosine 1-phosphate receptor 2 (S1PR2) (33). The binding of bile acid to S1PR2 can exacerbate liver fibrosis severity by activating the extracellular signal-regulated protein kinase 1/2 (ERK1/2) signaling pathway. Moreover, bile acids are directly involved in disrupting the cell membrane by activating the p38 MAPK, nuclear factor-kappaB (NF-κB), and phospholipase A2 pathways, generating much more toxic reactive oxygen species and therefore inducing liver fibrosis. In agreement with previous molecular mechanisms, elevated TBA has been found to be a marker of liver fibrosis progression among patients with chronic liver diseases (34). In a clinical study with 328 patients with chronic hepatitis B infection, TBA presented significant positive correlations with biopsy-proven significant fibrosis stage (r=0.38, P<0.001) (35). Similarly, a recent study also reported a dose-dependent relationship between lesions of steatosis-related fibrosis status confirmed by histology and serum TBA levels determined by liquid chromatograph-mass spectrometer (36). Our results additionally highlighted the potential role of monitoring dynamic changes in TBA for predicting fibrosis progression.
Regarding the association between changes in hepatic steatosis and fibrosis, the current study proved a significant association between steatosis improvement and fibrosis regression at 24 weeks in MASLD patients, which was in accordance with previous studies (37,38). Significantly, this study is the first to further explore the association between the loss of sustained steatosis response by MRI-PDFF and the loss of sustained fibrosis response by 2D-SWE. However, we were not able to identify significant association at 48 weeks. Although previously underappreciated, this phenomenon may be attributed to that resolution and progression of hepatic steatosis and fibrosis represent a complex interplay of genetic, environmental, and intrinsic microbial factors (39). Previous researches have revealed that the majority (approximately 80%) of MASLD patients experienced gradual fibrosis progression over time, and simple steatosis typically experienced 1 stage of fibrosis progression over an average of 14.3 years (40). And the number of MASLD patients with LSM ≥6.4 kpa who completed the 48-week follow-up was too small in this study to draw meaningful conclusions regarding fibrosis regression. Therefore, further investigations are warranted to explore this relationship in studies with larger sample sizes and longer follow-up.
This study has several strengths. Our results were obtained from a well-established prospective cohort with MRI-PDFF and SWE regular monitoring, first exploring the phenomenon of loss of response in MASLD long-term management. To identify predictors of loss of response, we also documented data on daily dietary habits and exercise, which can further help to classify potential confounders. Several limitations also exist in the current research. Inflammatory mediators, including interleukin levels, lack measurements. The other liver injury lesions, such as ballooning, were not included in this analysis. Part of the natural regression of steatosis was not distinguished from the lost response. The relatively small sample size and limited number of events per variable constrain the statistical power of the logistic regression analysis and the robustness of the findings (41), particularly in the assessment of subgroup liver fibrosis efficacy. Moreover, the excellent AUC values are based on separation between mild, significant, advanced fibrosis, the relevance of change of SWE is not validated so any claims regarding this must be done with great caution and those with such a significant change in fibrosis stage based on SWE during a short study should be interpreted with the greatest of caution and need more validation. Although our subjects were included in the largest tertiary center in southern China, the generalizability would inevitably be attenuated.
Conclusions
The gap between therapeutic needs and outcomes for MASLD continues to expand because no effective pharmacracy interventions have been widely approved. Current guidelines/consensuses provide unmeet instruction to clinicians for treating patients with loss of response. The current analysis indicates that patients who achieve an MRI-PDFF response but with IR should undergo intensive lifestyle interventions, as monitoring TBA levels and lowering it may benefit from delaying fibrosis progression. As MASLD comprises a heterogeneous pathology mechanism (42), precise treatment or treatment of multiple targets remains necessary.
Acknowledgments
We are grateful to Professor Aihua Lin in School of Public Health, Sun Yat-sen University for her assistance in statistical analysis of this study.
Funding: This work was supported by
Footnote
Reporting Checklist: The authors have completed the STROBE reporting checklist. Available at https://hbsn.amegroups.com/article/view/10.21037/hbsn-23-393/rc
Data Sharing Statement: Available at https://hbsn.amegroups.com/article/view/10.21037/hbsn-23-393/dss
Peer Review File: Available at https://hbsn.amegroups.com/article/view/10.21037/hbsn-23-393/prf
Conflicts of Interest: All authors have completed the ICMJE uniform disclosure form (available at https://hbsn.amegroups.com/article/view/10.21037/hbsn-23-393/coif). The authors have no conflicts of interest to declare.
Ethical Statement: The authors are accountable for all aspects of the work in ensuring that questions related to the accuracy or integrity of any part of the work are appropriately investigated and resolved. The study was conducted in accordance with the Declaration of Helsinki (as revised in 2013). The study protocol was approved by the Ethics Committee of The First Affiliated Hospital, Sun Yat-sen University {[2014]112}. All participants provided signed informed consent prior to enrollment.
Open Access Statement: This is an Open Access article distributed in accordance with the Creative Commons Attribution-NonCommercial-NoDerivs 4.0 International License (CC BY-NC-ND 4.0), which permits the non-commercial replication and distribution of the article with the strict proviso that no changes or edits are made and the original work is properly cited (including links to both the formal publication through the relevant DOI and the license). See: https://creativecommons.org/licenses/by-nc-nd/4.0/.
References
- Rinella ME, Lazarus JV, Ratziu V, et al. A multisociety Delphi consensus statement on new fatty liver disease nomenclature. J Hepatol 2023;79:1542-56. [Crossref] [PubMed]
- Younossi ZM, Golabi P, Paik JM, et al. The global epidemiology of nonalcoholic fatty liver disease (NAFLD) and nonalcoholic steatohepatitis (NASH): a systematic review. Hepatology 2023;77:1335-47. [Crossref] [PubMed]
- Estes C, Anstee QM, Arias-Loste MT, et al. Modeling NAFLD disease burden in China, France, Germany, Italy, Japan, Spain, United Kingdom, and United States for the period 2016-2030. J Hepatol 2018;69:896-904. [Crossref] [PubMed]
- Wong VW, Chan WK, Chitturi S, et al. Asia-Pacific Working Party on Non-alcoholic Fatty Liver Disease guidelines 2017-Part 1: Definition, risk factors and assessment. J Gastroenterol Hepatol 2018;33:70-85. [Crossref] [PubMed]
- Chitturi S, Wong VW, Chan WK, et al. The Asia-Pacific Working Party on Non-alcoholic Fatty Liver Disease guidelines 2017-Part 2: Management and special groups. J Gastroenterol Hepatol 2018;33:86-98. [Crossref] [PubMed]
- Cusi K, Isaacs S, Barb D, et al. American Association of Clinical Endocrinology Clinical Practice Guideline for the Diagnosis and Management of Nonalcoholic Fatty Liver Disease in Primary Care and Endocrinology Clinical Settings: Co-Sponsored by the American Association for the Study of Liver Diseases (AASLD). Endocr Pract 2022;28:528-62. [Crossref] [PubMed]
- European Association for the Study of the Liver (EASL), European Association for the Study of Diabetes (EASD), European Association for the Study of Obesity (EASO). EASL-EASD-EASO Clinical Practice Guidelines for the management of non-alcoholic fatty liver disease. J Hepatol 2016;64:1388-402. [Crossref] [PubMed]
- Qu Y, Li M, Hamilton G, et al. Diagnostic accuracy of hepatic proton density fat fraction measured by magnetic resonance imaging for the evaluation of liver steatosis with histology as reference standard: a meta-analysis. Eur Radiol 2019;29:5180-9. [Crossref] [PubMed]
- Stine JG, Munaganuru N, Barnard A, et al. Change in MRI-PDFF and Histologic Response in Patients With Nonalcoholic Steatohepatitis: A Systematic Review and Meta-Analysis. Clin Gastroenterol Hepatol 2021;19:2274-2283.e5. [Crossref] [PubMed]
- Xiao G, Zhu S, Xiao X, et al. Comparison of laboratory tests, ultrasound, or magnetic resonance elastography to detect fibrosis in patients with nonalcoholic fatty liver disease: A meta-analysis. Hepatology 2017;66:1486-501. [Crossref] [PubMed]
- Yuan MY, He JR, Chen NN, et al. Validity and Reproducibility of a Dietary Questionnaire for Consumption Frequencies of Foods during Pregnancy in the Born in Guangzhou Cohort Study (BIGCS). Nutrients 2016;8:454. [Crossref] [PubMed]
- Yang Y, He M, Pan X. China Food Composition Tables: Standard Edition. 6th ed. Beijing: Peking University Medical Press; 2018.
- Park S, Buranakitjaroen P, Chen CH, et al. Expert panel consensus recommendations for home blood pressure monitoring in Asia: the Hope Asia Network. J Hum Hypertens 2018;32:249-58. [Crossref] [PubMed]
- Shao C, Ye J, Li F, et al. Early Predictors of Cardiovascular Disease Risk in Nonalcoholic Fatty Liver Disease: Non-obese Versus Obese Patients. Dig Dis Sci 2020;65:1850-60. [Crossref] [PubMed]
- Li R, Liao XH, Ye JZ, et al. Association of keratin 8/18 variants with non-alcoholic fatty liver disease and insulin resistance in Chinese patients: A case-control study. World J Gastroenterol 2017;23:4047-53. [Crossref] [PubMed]
- American Diabetes Association. 2. Classification and Diagnosis of Diabetes: Standards of Medical Care in Diabetes-2020. Diabetes Care 2020;43:S14-31. [Crossref] [PubMed]
- Dong Z, Luo Y, Zhang Z, et al. MR quantification of total liver fat in patients with impaired glucose tolerance and healthy subjects. PLoS One 2014;9:e111283. [Crossref] [PubMed]
- Gu J, Liu S, Du S, et al. Diagnostic value of MRI-PDFF for hepatic steatosis in patients with non-alcoholic fatty liver disease: a meta-analysis. Eur Radiol 2019;29:3564-73. [Crossref] [PubMed]
- Ye J, Wang W, Feng S, et al. Precise fibrosis staging with shear wave elastography in chronic hepatitis B depends on liver inflammation and steatosis. Hepatol Int 2020;14:190-201. [Crossref] [PubMed]
- Imajo K, Honda Y, Kobayashi T, et al. Direct Comparison of US and MR Elastography for Staging Liver Fibrosis in Patients With Nonalcoholic Fatty Liver Disease. Clin Gastroenterol Hepatol 2022;20:908-917.e11. [Crossref] [PubMed]
- Chinese Nutrition Society. Chinese Dietary Reference Intakes (2013). Beijing: Science Press; 2013:660.
- Waxman A. WHO global strategy on diet, physical activity and health. Food Nutr Bull 2004;25:292-302. [Crossref] [PubMed]
- Younossi ZM, Corey KE, Lim JK. AGA Clinical Practice Update on Lifestyle Modification Using Diet and Exercise to Achieve Weight Loss in the Management of Nonalcoholic Fatty Liver Disease: Expert Review. Gastroenterology 2021;160:912-8. [Crossref] [PubMed]
- Ryu S, Chang Y, Kim DI, et al. gamma-Glutamyltransferase as a predictor of chronic kidney disease in nonhypertensive and nondiabetic Korean men. Clin Chem 2007;53:71-7. [Crossref] [PubMed]
- Osaka T, Hashimoto Y, Hamaguchi M, et al. Nonalcoholic fatty liver disease remission in men through regular exercise. J Clin Biochem Nutr 2018;62:242-6. [Crossref] [PubMed]
- Wang J, Qi Y, Xin Z, et al. Isotemporal substitution of different behaviour patterns with the presence of MAFLD in Chinese adults. Liver Int 2022;42:2683-95. [Crossref] [PubMed]
- Chinese Multidisciplinary Expert Consensus on the Diagnosis and Treatment of Hyperuricemia and Related Diseases. Chin Med J (Engl) 2017;130:2473-88. [Crossref] [PubMed]
- Weng J, Ji L, Jia W, et al. Standards of care for type 2 diabetes in China. Diabetes Metab Res Rev 2016;32:442-58. [Crossref] [PubMed]
- Grundy SM, Stone NJ, Bailey AL, et al. 2018 AHA/ACC/AACVPR/AAPA/ABC/ACPM/ADA/AGS/APhA/ASPC/NLA/PCNA Guideline on the Management of Blood Cholesterol: A Report of the American College of Cardiology/American Heart Association Task Force on Clinical Practice Guidelines. J Am Coll Cardiol 2019;73:e285-350. [Crossref] [PubMed]
- Romero-Gómez M, Zelber-Sagi S, Trenell M. Treatment of NAFLD with diet, physical activity and exercise. J Hepatol 2017;67:829-46. [Crossref] [PubMed]
- Ye J, Hu X, Wu T, et al. Insulin resistance exhibits varied metabolic abnormalities in nonalcoholic fatty liver disease, chronic hepatitis B and the combination of the two: a cross-sectional study. Diabetol Metab Syndr 2019;11:45. [Crossref] [PubMed]
- Luo L, Aubrecht J, Li D, et al. Assessment of serum bile acid profiles as biomarkers of liver injury and liver disease in humans. PLoS One 2018;13:e0193824. [Crossref] [PubMed]
- Thomas C, Pellicciari R, Pruzanski M, et al. Targeting bile-acid signalling for metabolic diseases. Nat Rev Drug Discov 2008;7:678-93. [Crossref] [PubMed]
- Wang X, Xie G, Zhao A, et al. Serum Bile Acids Are Associated with Pathological Progression of Hepatitis B-Induced Cirrhosis. J Proteome Res 2016;15:1126-34. [Crossref] [PubMed]
- Xie R, Li J, Zhang H, et al. Total serum bile acids predict therapy for HBeAg-negative chronic hepatitis B patients with borderline ALT and high HBV DNA. J Infect Dev Ctries 2022;16:1336-42. [Crossref] [PubMed]
- Caussy C, Hsu C, Singh S, et al. Serum bile acid patterns are associated with the presence of NAFLD in twins, and dose-dependent changes with increase in fibrosis stage in patients with biopsy-proven NAFLD. Aliment Pharmacol Ther 2019;49:183-93. [Crossref] [PubMed]
- Tamaki N, Munaganuru N, Jung J, et al. Clinical utility of 30% relative decline in MRI-PDFF in predicting fibrosis regression in non-alcoholic fatty liver disease. Gut 2022;71:983-90. [Crossref] [PubMed]
- Huang DQ, Sharpton SR, Amangurbanova M, et al. Clinical Utility of Combined MRI-PDFF and ALT Response in Predicting Histologic Response in Nonalcoholic Fatty Liver Disease. Clin Gastroenterol Hepatol 2023;21:2682-2685.e4. [Crossref] [PubMed]
- Wree A, Broderick L, Canbay A, et al. From NAFLD to NASH to cirrhosis-new insights into disease mechanisms. Nat Rev Gastroenterol Hepatol 2013;10:627-36. [Crossref] [PubMed]
- Singh S, Allen AM, Wang Z, et al. Fibrosis progression in nonalcoholic fatty liver vs nonalcoholic steatohepatitis: a systematic review and meta-analysis of paired-biopsy studies. Clin Gastroenterol Hepatol 2015;13:643-54.e1-9; quiz e39-40.
- van Smeden M, de Groot JA, Moons KG, et al. No rationale for 1 variable per 10 events criterion for binary logistic regression analysis. BMC Med Res Methodol 2016;16:163. [Crossref] [PubMed]
- Ye J, Zhuang X, Li X, et al. Novel metabolic classification for extrahepatic complication of metabolic associated fatty liver disease: A data-driven cluster analysis with international validation. Metabolism 2022;136:155294. [Crossref] [PubMed]